To What Extent Do Musculoskeletal Ultrasound Biomarkers Relate to Pain, Flexibility, Strength, and Function in Individuals With Chronic Symptomatic Achilles Tendinopathy?
- 1Faculty of Medicine, Université de Montréal, Montreal, QC, Canada
- 2Centre for Interdisciplinary Research in Rehabilitation of Greater Montreal (CRIR), Montreal, QC, Canada
- 3Institut de recherche Robert-Sauvé en santé et en sécurité du travail (IRSST), Montreal, QC, Canada
- 4Centre de recherche de l'Hôpital Maisonneuve-Rosemont (CRHMR), Montreal, QC, Canada
Introduction: Achilles tendinopathy (AT) is a chronic musculoskeletal pathology best evaluated by ultrasound imaging. This cross-sectional study aimed at better understanding the relationship between musculoskeletal ultrasound biomarkers (MUBs) of Achilles tendon and localized pain, ankle flexibility, ankle strength, and functional abilities.
Method: Forty-one participants with unilateral midportion chronic AT had their tendon images analyzed bilaterally in the longitudinal and transverse planes. The Victorian Institute of Sport Assessment-Achilles questionnaire (VISA-A) and Lower Extremity Functional Scale (LEFS) assessed pain and function, respectively, during standing and walking-related activities. Ankle flexibility was evaluated by weight-bearing lunge tests, while ankle isometric peak strength was measured using an instrumented dynamometer. Achilles tendon ultrasonographic images were analyzed using geometric (thickness), composition (echogenicity), and texture (homogeneity) MUBs. Discriminative validity was evaluated using paired Student's t-tests to compare MUBs between symptomatic and asymptomatic sides. Predictive validity was evaluated by computing the Pearson product-moment correlations coefficient between MUBs and pain, ankle flexibility, ankle strength, and function.
Results: Significant differences were found in MUBs between the symptomatic and asymptomatic sides, confirming the discriminative validity of the selected MUBs. On the symptomatic side, thickness was found 29.9% higher (p < 0.001), echogenicity 9.6% lower (p < 0.001), and homogeneity 3.8% higher (p = 0.001) when compared with the asymptomatic side. However, predictive validity was scarcely confirmed, as most of the correlation coefficients were found negligible for the associations investigated between MUBs with localized pain, ankle flexibility, strength, and function. Only 14 statistically significant low to moderate associations were found, with negative and positive correlations ranging between −0.31 and −0.55 and between 0.34 and 0.54, respectively.
Discussion: Musculoskeletal ultrasound biomarkers have a clinical utility in visualizing in vivo tendon integrity and diagnosing AT. MUBs should be valued as part of a comprehensive neuro-musculoskeletal assessment as they complement pain, flexibility, strength, and function measures. Altogether, they may inform the development and monitoring of a personalized rehabilitation treatment plan.
Introduction
Achilles tendinopathy (AT) is a common musculoskeletal pathology that affects the strongest and largest tendon of the human body (1–3). AT generally affects either the midportion of the Achilles tendon or its enthesis on the calcaneus among both sedentary and athletic individuals (1–4). The prevalence of AT increases with age, and AT mostly affects males between 30 and 50 years of age. Although, the precise etiology of AT remains uncertain, the risk of injury is mainly associated with a maladaptive response to overstimulation caused by repetitive plantarflexion and high forces transmitted through the tendon during functional activities involving plantarflexion (5). Other factors such as the presence of common chronic diseases (i.e., diabetes, rheumatoid arthritis, or hypercholesterolaemia), training errors, cold environments, or the use of specific medications (i.e., corticosteroids, fluoroquinolone, statins) may also increase the risk of AT (4, 6, 7). AT-related symptoms typically include pain at rest, pain during or after physical activities, and a feeling of stiffness of the Achilles tendon after a period of immobility. AT main impairments include reduced flexibility of the triceps surae muscles (i.e., gastrocnemius and soleus) and a decrease in both force and endurance of triceps surae muscles (mostly the soleus) (5, 8, 9). These symptoms and impairments typically lead to functional limitations when walking, running, using stairs, and jumping, leading to decreased social participation and quality of life (4, 10, 11).
In chronic AT, episodes of excessive mechanical stress cause pathological changes in the tendon that are visible and distinctive on ultrasound images (4, 12, 13). Affected tendons typically present abnormal collagen and extracellular matrix composition and structure (14). These abnormalities of the Achilles tendon can be quantified by musculoskeletal ultrasound biomarkers (MUBs), such as geometric (thickening), composition (hypoechogenic), or texture (increased homogeneity) dimensions (15–18). MUBs extracted from ultrasound imaging have good reliability when characterizing the biological integrity of the Achilles tendon (19). Furthermore, MUBs allow differentiating between several pathologies (e.g., partial tear, bursitis, peritendinitis) when clinical evaluation is not conclusive (4). Therefore, MUBs are tools frequently used in both clinical and research settings when characterizing the Achilles tendon integrity (20–24).
Interestingly, bodies of evidence are conflicting regarding associations between MUBs and pain, flexibility, strength, and function (25–32). Some authors found a lack of association between anatomopathology and symptom severity in cross-sectional studies (26, 27) or limited structural improvement of the tendon despite clinical improvement in terms of pain and function among individuals with midportion AT during prospective studies (33–35). Inversely, some authors found a significant association between tendon thickness, hypoechogenicity, and pain among cross-sectional studies (25) and prospective studies (36, 37). Consequently, there is no consensus regarding the association between MUBs and clinical outcomes among individuals with AT.
The main objective of the study was to gain knowledge and a better understanding of the relationship between geometric (i.e., thickness), composition (i.e., echogenicity), and texture (i.e., homogeneity) MUBs with localized pain, ankle flexibility, ankle strength, and standing-related functional abilities (e.g., walking, hopping, stairs, sports) among individuals with symptomatic midportion unilate×|<ral AT. More specifically, in terms of discriminative validity, MUBs of symptomatic Achilles tendons were generally expected to appear on images as thicker (i.e., increased thickness), darker (i.e., reduced echogenicity), and smoother (i.e., increased homogeneity) in comparison with the asymptomatic Achilles tendons (15). Moreover, in terms of predictive validity, absolute (i.e., symptomatic tendon) or relative (i.e., symptomatic/non-symptomatic tendon difference) MUBs were expected to be, at least, moderately associated with localized pain, ankle flexibility, strength impairments, and functional disabilities.
Method
Study Design
This validity study builds on a previously published one that proposed a minimal data set of MUBs to characterize Achilles tendon health (15). Additional clinical and laboratory measures, which may theoretically relate to MUBs, are now examined to test the hypotheses formulated above.
Participants
A group of 41 individuals with unilateral midportion chronic AT participated in the study (Table 1). Out of these 41 individuals, additional measures [i.e., Lower Extremity Functional Scale (LEFS) score and peak isometric ankle strength] were collected for the last 20 participants, as the research protocol was updated. Hence, these last 20 participants form a distinct subset of participants (i.e., subgroup). To be included in the study, potential participants had to report unilateral and localized pain in the Achilles tendon for more than 3 months (6), experience pain on palpation of the midportion of the Achilles tendon, and achieve a score below 90 out of 100 on the Victorian Institute of Sport Assessment-Achilles Questionnaire (VISA-A) (38). Potential participants who reported bilateral pain during activities had a body mass index (BMI) >30 kg/m2 and reported a history of Achilles tendon rupture, were diagnosed with a metabolic, neurologic, or systemic inflammatory disease, or had received any type of injection in the Achilles tendon in the past year were excluded.
Clinical Evaluations
Clinical evaluations were conducted by two trained experienced physiotherapists (ML and MJN) using a standardized data collection protocol.
Sociodemographic, Anthropometric, Pain, and Functional Assessments
Basic sociodemographic (i.e., age, sex) and anthropometric data (i.e., height, weight) were collected first before documenting AT-related information (e.g., the side affected, time since the first symptom, etc.,). The participants then completed one or two patient-reported outcome measures depending on when they entered the study. All the participants filled out the VISA-A (39). This questionnaire includes eight questions targeting three dimensions: localized pain in the Achilles tendon, function in daily life, and participation in sports activities (38–40). Questionnaire scores range from 0 to 100, with a low score indicating greater severity of the impact of the AT. The questionnaire is available in English and French, and it is reliable, valid, and sensitive to change with a minimal clinically important difference (MCID) of between 6 and 20 points (38, 41). The LEFS (42) questionnaire was only completed by the participants in the subgroup. The LEFS assesses the function of individuals with orthopedic disorders affecting their lower extremities, such as AT (42). The LEFS includes 20 questions measuring the level of difficulty encountered when performing activities of daily living and sports. The LEFS has a maximum score of 80, with a higher score confirming a higher functional level (42). The LEFS, which is also available in English and French, is reliable, valid, and sensitive to changes with a MCID of 9–12 points (41). The VISA-A and LEFS were completed either on paper or electronically via the Lime Survey® platform in the choice of language (French or English) of the participants.
Ankle Flexibility
Mono- and bi-articular muscle flexibility tests of the ankle were performed by the front-limb weight-bearing lunge test to assess the soleus and the back-limb weight-bearing lunge test with the knee extended to assess the flexibility of the gastrocnemius muscles (43, 44). For these two tests, the distance between the tip of the hallux and the wall was measured in cm. A reduction in flexibility of the soleus would translate to a decrease in the distance between the wall and the hallux of the front limb, whereas, reduced flexibility of the gastrocnemius muscles would translate to a decrease in the distance between the wall and the hallux of the back limb.
Isometric Strength
Peak isometric ankle strength, assessed exclusively in the subgroup, was measured using a Biodex instrumented dynamometer (Biodex Medical Systems, Shirley, NY, United States). The participants sat in a multi-position adjustable chair, with their hip flexed to 135°, knee extended, and the ankle firmly attached to a custom-made boot attached to the dynamometer to isolate ankle movement and prevent the heel from lifting during plantarflexion (45). The ankle was positioned at 10° plantarflexion during the isometric strength assessment (46). The physiotherapist (ML), assisted by a physiotherapy student (SP), instructed the participants to gradually contract their plantarflexor muscles up to their peak strength then hold the contraction for about 2 s (a total of ~5 s of contraction), while standard verbal encouragement was provided to ensure maximal effort throughout the tests (47, 48). Two trials were recorded, and a third one was recorded whenever the difference was >10% between the first two trials. A 1-min break was allowed between each trial. Each participant familiarized themselves with the dynamometer prior to the assessment (47, 49). The same process was applied for measuring isometric dorsiflexion force, but with the foot at 20° plantarflexion. The mean peak moment was computed for plantarflexion and dorsiflexion separately for both the symptomatic and asymptomatic sides. A dynamometer provided reliable and valid measures to characterize the strength-generating capability at the ankle (50, 51), and to differentiate individuals with good tendon health from those with AT (21, 52).
Musculoskeletal Ultrasound Imaging
Image-Acquisition
All the ultrasound images of the Achilles tendon were acquired in brightness mode using a 5–12 MHz linear array transducer with a 5-cm wide footprint connected to an HD 11XE 1.0.6 ultrasonography system (Phillips Medical Systems, Bothell, WA, United States). A previously described standardized protocol (15, 19) was used for image acquisition. All parameters affecting image quality (i.e., probe frequency set at 12 MHz; depth = 2 cm; gain = 85; unique focus zone adjusted to 0.5 cm at the AT level; neutral time gain compensation) remained constant during each data collection session and across the participants. In addition, care was taken to align the transducer parallel to fiber orientation to minimize anisotropy. The most painful region along the posterior aspect of the symptomatic tendon was first located by palpation before its location was marked on the skin and mirrored on the asymptomatic side. The probe was then positioned at the marked site to view the Achilles tendon fibers in the longitudinal and transverse planes, respectively (Figure 1A). Three images were acquired in both the longitudinal and transverse planes (n = 6 images per side). The probe was removed after the recording of each image and always repositioned at the marked site thereafter.
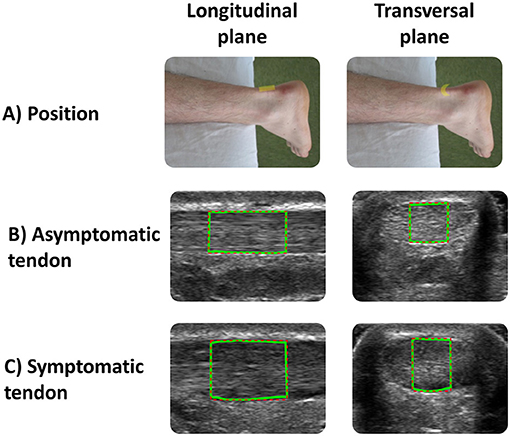
Figure 1. (A) Positions of the transducer in the longitudinal and transverse planes used to acquire asymptomatic and symptomatic Achilles tendon ultrasound images. The green lines correspond to the region of interest (ROI) used for data analysis for (B) asymptomatic and (C) symptomatic tendon.
Image-Analysis
All the images were analyzed using a custom interactive 2D viewing and image analysis program developed by the research team with Image Processing Toolbox™ from MATLAB (MathWorks Inc., Natick, MA, United States), as used in previous studies (19, 53). Using this program, a region of interest (ROI) was manually outlined within the hyperechoic margins of the tendon (i.e., circumferential epitenon) (Figure 2). A semi-automatic tracing procedure then selected the lateral margins of the 1-cm and 0.5-cm wide ROIs in the longitudinal and transverse planes, respectively (Figures 1B,C). In the transverse plane, this process also reduces lateral anisotropy and consequently improves the reliability of MUBs (53, 54). Based on the previously recommended minimal dataset organized around three dimensions (i.e., geometric, composition, and texture), MUBs were computed for each ROI taken in the longitudinal and transverse planes (i.e., mean thickness, echogenicity, and homogeneity at 90° in the longitudinal plane and multidirectional mean in the transverse plane). MUB values of the three measurements were averaged for each plane. Additional details on the various measures are available elsewhere (19).
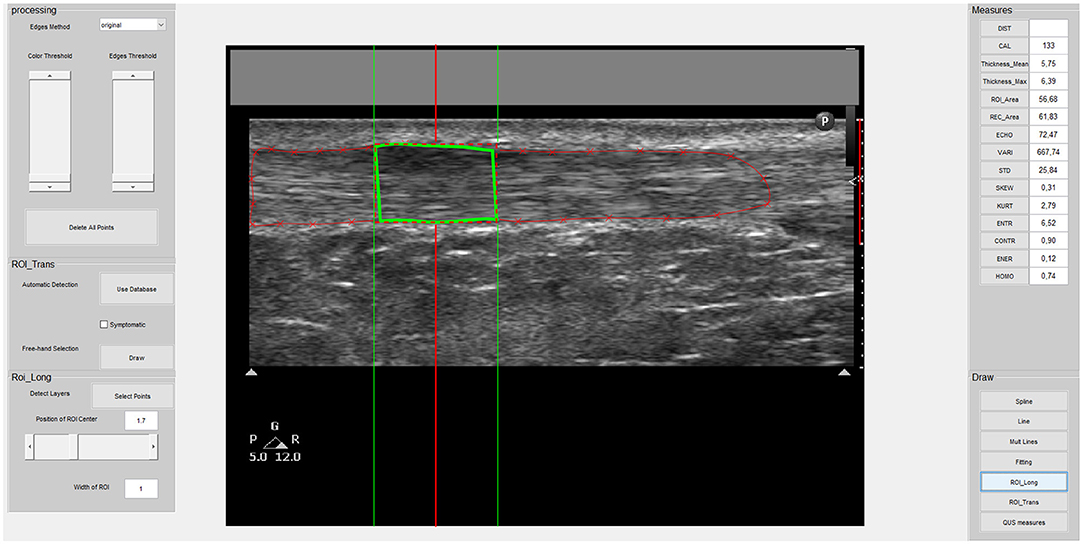
Figure 2. Screenshot of the custom-made program interface used to select the ROI and acquire musculoskeletal ultrasound biomarker (MUB) measures of the Achilles tendon image. This is an example of a symptomatic tendon in the longitudinal plane with a 1-cm wide ROI.
Statistical Analyses
Descriptive statistics (e.g., mean, SD, proportion, range) synthesized the demographics, and the anthropometric clinical and laboratory outcomes. The normality of the distribution of outcomes was verified by the Shapiro–Wilk test and using a graphical method. Differences between the main group (n = 41) and the subgroup (n = 20) demographic, pain, and functional measures were compared by bivariate independent Student's t-test for parametric data and the chi-squared test for non-parametric data. Differences between the asymptomatic and symptomatic sides for clinical (i.e., flexibility and strength) and ultrasound (i.e., mean thickness, echogenicity, and homogeneity) measures were assessed by independent paired Student's t-tests and Wilcoxon signed-rank tests for normally and non-normally distributed data, respectively. Effect sizes were also computed using Hedge's g (55) to determine the absolute magnitude of the effect sizes: >0.2 was considered small, >0.5 was considered medium, and >0.8 was considered large (56). Thereafter, a difference index (DI) was computed to assess the relative difference between the symptomatic and asymptomatic sides for all clinical and ultrasound measures using Equation 1:
Last, the association between the MUBs and the absolute (i.e., symptomatic side) and relative (i.e., DI) measures of localized pain (i.e., VISA-A), ankle flexibility and strength impairments, and functional disabilities (i.e., VISA-A and LEFS) were assessed using Pearson product-moment correlation and Spearman rank-order correlation for normally and non-normally distributed data, respectively. The strength of correlation coefficients was considered negligible between 0 and .3, low between 0.3 and 0.5, moderate between 0.5 and 0.7, and high between 0.7 and 0.9 (57). The threshold for statistical significance was set at 0.05 for all statistical analyses carried out with the SPSS v25 software.
Results
Characteristics of Participants
A summary of the characteristics of the participants and scores achieved on the VISA-A and LEFS questionnaires are presented in Table 1. Participant characteristics were not significantly different (p = 0.147 to 0.949) between the main group and the subgroup.
Clinical Measures
A summary of the functional measures is presented in Table 1, whereas, the clinical measures and MUBs are summarized in Table 2. Only flexibility measures for the soleus revealed a significant (p = 0.002) but the small between-side difference (Hedge's g of −0.23) in soleus flexibility. The soleus on the symptomatic side was 7.3% less flexible than the one on the asymptomatic side. The flexibility of the gastrocnemius (p = 0.245) and the isometric strength of the ankle plantarflexors (p = 0.182) and dorsiflexors (p = 0.52) were similar between the asymptomatic and symptomatic sides.
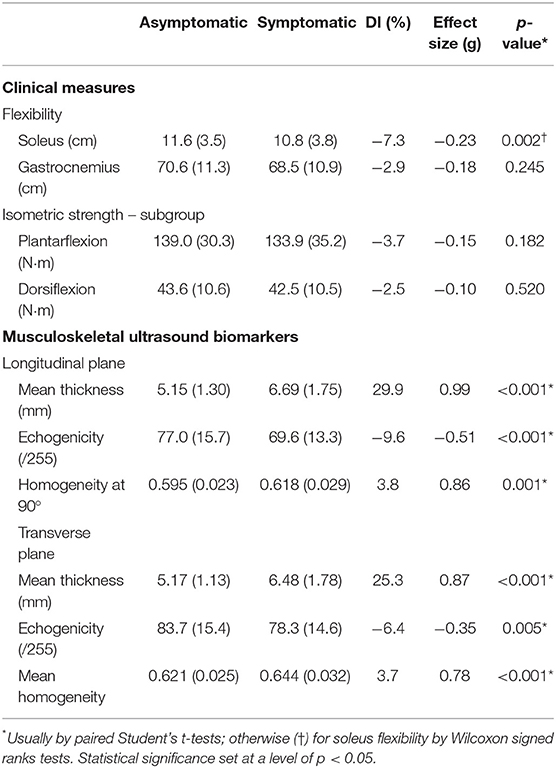
Table 2. Mean (SD) clinical measures, musculoskeletal ultrasound biomarkers (MUBs), and difference index (DI) between sides.
Musculoskeletal Ultrasound Biomarkers
A summary of the MUBs is presented in Table 2. The mean thickness of the Achilles tendon revealed a significant (p < 0.001) and large between-side difference (g = 0.99 and 0.87), reaching +29.9 and +25.3% for the symptomatic tendon when compared with the asymptomatic tendon in the longitudinal and transverse planes, respectively. The echogenicity of the tendon revealed a significant (p < 0.001 and p = 0.005) and medium to small between-side difference (g = −0.51 and −0.35), reaching −9.6 and −6.4% for the symptomatic tendon when compared with the asymptomatic tendon in the longitudinal and transverse planes, respectively. The homogeneity of the tendon revealed a significant (p < 0.001 and p < 0.001) and large to medium between-side difference (g = 0.86 and 0.78), reaching 3.8 and 3.7% for the symptomatic tendon when compared with the asymptomatic tendon in the longitudinal and transverse planes, respectively.
Associations Between MUBs and Other Measures
A summary of the associations between the MUBs and clinical and functional measures is presented in Table 3 and Figure 3. Overall, the correlation coefficients were found to be negligible for most of the associations investigated. Only few statistically significant low to moderate correlations were found (n = 14, negative and positive correlations ranging between −0.31 and −0.55 and between 0.34 and 0.54, respectively). Among the clinical measures, low correlations were found between the flexibility of the gastrocnemius in both planes and the absolute thickness-Sympt and echogenicity-Sympt. Also, low to moderate correlations were found between the ankle plantarflexor strength-DI and the echogenicity-DI in the longitudinal plane and between the ankle dorsiflexor strength-Sympt and the mean thickness in both planes. Among the pain and functional measures, the mean thickness of the symptomatic tendons in both planes was consistently correlated with questions #7 and #8 of the VISA-A, suggesting higher limitations in sports level and time as tendon thickness increases. For these two questions, albeit not as consistently correlated as for thickness, few echogenicity and homogeneity measures were also found to be significantly associated. In the longitudinal plane, homogeneity and question #5 – painful heel raise as well as between echogenicity and question #6 – pain during hopping. No significant correlations (i.e., low, moderate, or high) were observed between the total VISA-A score and MUBs (Figure 3), or between the LEFS questionnaire and the MUBs.
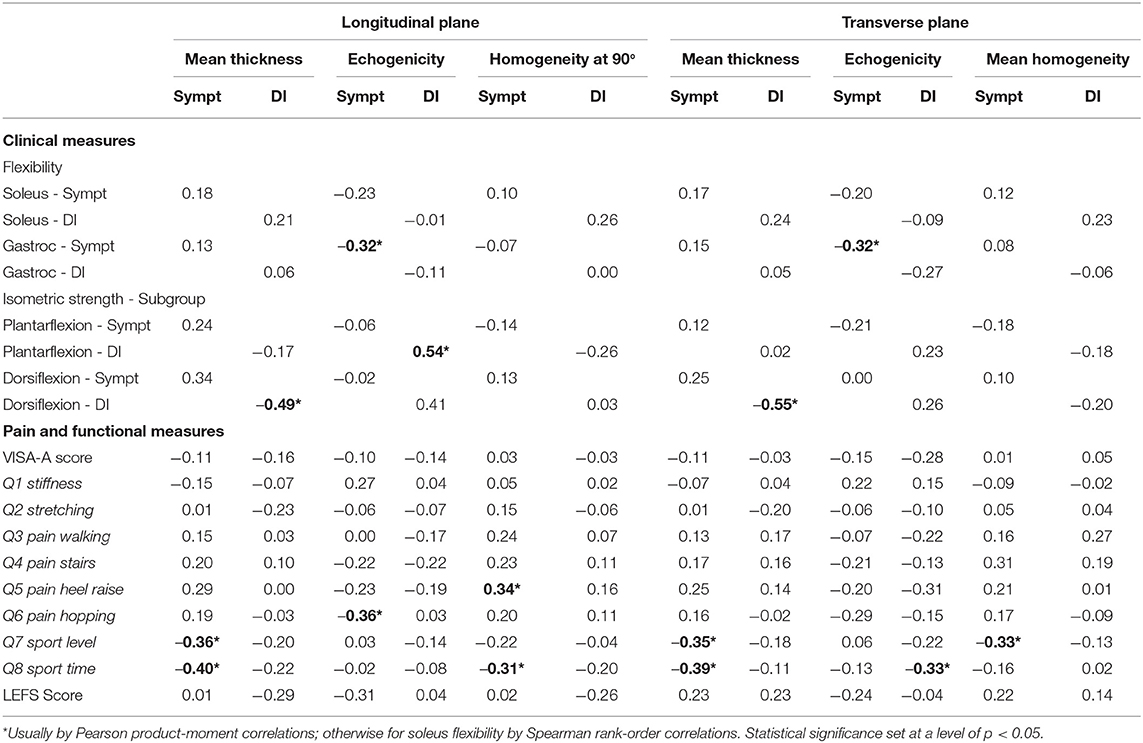
Table 3. Associations between MUBs and clinical measures for the symptomatic (Sympt) side and DI separately.
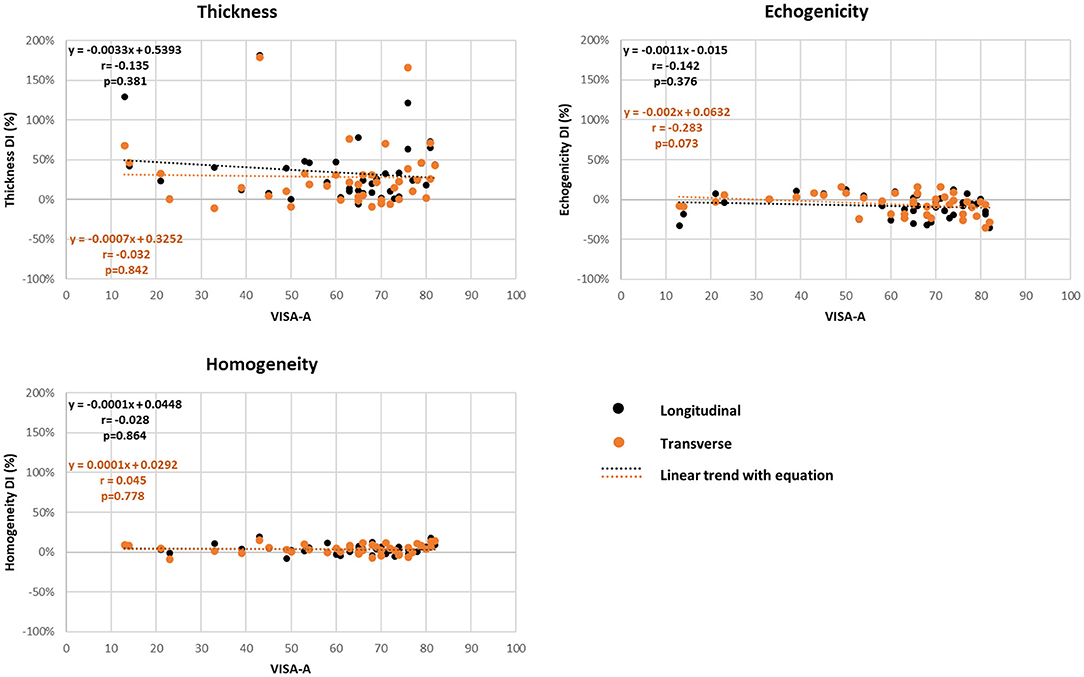
Figure 3. Correlation (r) of ultrasound biomarker difference index (DI) measures and Victorian Institute of Sport Assessment-Achilles Questionnaire (VISA-A) total score (Pearson product-moment test).
Discussion
In line with previous research studies, this one further explored the discriminative ability of MUBs and their predictive validity with regard to localized tendon pain, ankle flexibility, ankle strength, and standing-related functional abilities among individuals with unilateral symptomatic AT. The results confirm that the discriminative validity of MUBs is high between the symptomatic and asymptomatic sides, whereas, the predictive validity of MUBs with patient-reported outcome measures and clinically related outcome measures are limited and inconsistent across the assessed clinical outcomes (i.e., pain, flexibility, strength, and functional abilities).
Discriminative Validity
In agreement with the first hypothesis, significant differences were found in MUBs between the symptomatic and asymptomatic Achilles tendons among individuals with chronic symptomatic unilateral AT, confirming the capacity of the selected MUBs to discriminate a symptomatic tendon from an asymptomatic one. Geometric, composition, and texture MUBs change (mostly at the cellular and structural level) in response to localized tendon maladaptive changes as tendinopathy develops (58). In the symptomatic tendon, abnormal tenocyte morphology and changes in proteoglycan content typically increase bound water and tendon thickness in comparison with the asymptomatic tendon (59). In addition, hydrated components of the extracellular matrix increase, and fibrillar disorganization progressively develops, resulting in a reduced quantity of intact collagen fibers and, consequently, reduction in reflected ultrasounds, and production of a darker hypo-echogenic image (13). Finally, fibrillar disorganization triggers changes from type I collagen in favor of type II and III collagens and alters parallel fiber alignment, resulting in alteration of the typical white and gray striated tendon pattern and, thus, smoother texture (i.e., increased homogeneity) (60). Hence, as anticipated, based on previous studies (61–63), the MUBs of symptomatic Achilles tendons generally stood out on the images as being thicker, darker, and smoother in comparison with the asymptomatic Achilles tendons, showing high discriminative validity. These findings further support the conclusions of Matthews et al. (62) who mentioned that geometric and composition MUBs (i.e., thickness and echogenicity) represent key MUBs for assessing images of symptomatic Achilles tendons in clinical and research settings. These findings also reinforce the clinical utility of quantifying echogenicity, instead of only subjectively identifying the presence or absence of hypoechogenic areas (62, 64).
The findings of this study also address a concern raised by Matthews et al. (62) who confirmed the relevance of texture-related MUBs in characterizing tendon matrix changes, although, they are typically lacking in the literature. In that sense, the findings of this study confirm that homogeneity is a MUB that allows clinicians to quantify the fibrillary disorganization of collagen fibers (54) and, as such, may deserve additional attention in future studies. Conversely, the findings of this study provide indirect evidence for the use of a contrast measure as a MUB, since it stands as a relative mathematical inverse of homogeneity in second-order statistics (19). Finally, to build on this study confirming that MUBs are valuable in discriminating a symptomatic tendon from an asymptomatic one (i.e., first iteration), future studies could focus on the classification of symptomatic tendons into tendinopathy stages (i.e., second iteration) as previously attempted (20). In line with this idea, using emerging machine learning and deep-learning approaches may further facilitate collaborative image-based decision-making (65). Achieving such a milestone could add value to the use of MUBs to support the clinical decision-making process.
Predictive Validity
Contrary to the second hypothesis, the findings of this study highlight the fact that both absolute and relative MUBs have only negligible to low associations with localized tendon pain, ankle flexibility and strength, and functional abilities among individuals with chronic unilateral AT. In fact, it was anticipated that the increased thickness, reduced echogenicity, and increased homogeneity observed at the symptomatic tendon could lead to a better understanding of the vicious cycle in which localized tendon pain, reduced ankle flexibility and strength, and reduced functional abilities and sport performances interact (66). Hence, substantial uncertainty still exists with regard to the predictive validity of MUBs (29, 64), and their added value to support the development of a personalized rehabilitation intervention treatment plan remains controversial (67). Moreover, controversies still exist as to whether or not MUBs can change concurrently to favorable treatment outcomes, as well as how and to what extent they may do so (26, 34, 35, 68).
Regarding pain-related measures, further reflection is needed to explain why they are not, at least, moderately associated with current MUBs in individuals who have chronic unilateral AT. Part of the answer may relate to the increase in angiogenic growth factors, resulting in considerable neovascularization, particularly on the ventral side of the tendon, along with nerve ingrowths that have been proposed as the origin of tendinopathy-related nociceptive pain (69). These peripheral adaptations may increase sensitivity to chemical pain mediators and trigger an over-activation of nociceptors (70, 71). Hence, the inclusion of quantitative Doppler-related MUBs may become indispensable to best capture such adaptions in the future, while also reinforcing their relevance in composite ultrasonography-based scoring when coupled with geometric and composition measures (i.e., thickness and echogenicity) (20, 72). Part of the answer may also relate to sensitization of the central nervous system (i.e., central adaptations), commonly associated with chronic musculoskeletal impairments (73, 74), or psychological factors affecting pain (e.g., pain catastrophizing and kinesiophobia) (75–77), which can both evolve independently to peripheral tendon alterations characterized with MUBs. Another part of the answer may ultimately relate to coexisting plantaris tendinopathy and surrounding tissue alterations, as these conditions affect about one in every 10 individuals with confirmed midportion AT (78, 79).
As for flexibility, strength, and function-related measures, despite tendon changes and the presence of pain expected to alter the loading capacity of the Achilles tendon, only few low to moderate associations were found between these constructs. First, the flexibility of the gastrocnemius on the symptomatic side pointed out a low association between the changes in flexibility and extracellular matrix content (i.e., reduced echogenicity) in both planes. Those results highlight that tendinopathy-related alterations in the Achilles tendon, characterized via composition and texture MUBs, do not predict flexibility of the triceps surae muscles (i.e., gastrocnemius and soleus). Second, despite a potentially altered loading capacity highlighted with the MUBs, only the echogenicity-DI was associated with the isometric strength-generating capability of the plantarflexors-DI. Such lack of strong and numerous associations may be explained in part by the fact that no significant difference was revealed between the symptomatic and asymptomatic sides when generating plantarflexion strength. Moreover, it is also plausible that the load generated was insufficient to observe the anticipated difference and, in the future, may warrant greater loading (e.g., eccentric tests, heel raises, or hopping) to gain a better understanding of the association between MUBs and strength (4, 43, 80). In that sense, the Q5 pain heel raise on the VISA-A, which coupled heavy load concentric-eccentric contractions, was associated, to some extent, with MUBs. Similar results were observed in a recent cross-sectional study investigating runners with midportion AT, where no significant difference in strength and endurance was found between the symptomatic and asymptomatic sides, although, the symptomatic side showed a significant decrease in plantarflexor strength when compared with healthy matched controls (9). Finally, for the functional outcome measures, the Q6 sport level and Q7 sport time on the VISA-A were predicted, to some extent, by some absolute and relative MUBs. This remains plausible considering sport level and maximal sport time may be altered by the loading capacity of the tendon, which changes as the tendon is altered by tendinopathy. Altogether, these results point toward potential central neuroplastic adaptations changing sensory and motor representations in individuals with a chronic musculoskeletal condition, such as in the population of individuals with chronic AT recruited in this study, resulting in bilateral perceptual changes of body image and motor control (73, 81). Ultimately, these adaptations may even trigger structural and histologic changes in the asymptomatic contralateral tendon in individuals with unilateral tendinopathy, providing further evidence for the bilateral nature of tendinopathy (30, 82, 83).
On the whole, the complex interactions between tendon changes and localized tendon pain, ankle flexibility, and strength, and functional abilities among individuals with chronic unilateral AT most likely explain the associations found in this study and definitively warrant further examination (58). Meanwhile, MUBs should be used in conjunction with clinical findings to adjust treatment protocol and improve clinical outcomes (75). Hence, gathering additional information through comprehensive anamnesis and neuro-musculoskeletal assessment continues to be vital for informing rehabilitation professionals in clinical practice and research environments.
Limitations
Some limitations of this study warrant discussion. First, the relatively modest sample of participants (n = 41) with AT, which was further reduced by the fact that ankle strength and LEFS data were only available for about half of the sample (n = 20), may have reduced the statistical power, although, moderate-to-large effect sizes were found for most MUBs discriminating the symptomatic and asymptomatic tendons (first hypothesis). Moreover, such sample size may have influenced data distribution and distorted correlation coefficients (second hypothesis). Second, the relatively homogeneous sample of participants with chronic midportion tendinopathy added to the fact that no specific consideration into the possible co-existence of plantaris tendinopathy (e.g., differential diagnosis) was incorporated in the research protocol (84), calls for cautiousness concerning the generalization of the results of this study in the context of an acute or insertional AT. Further caution is also advised if attempting to generalize these findings to other tendons (e.g., patellar tendon, supraspinatus tendon). Third, the fact that the asymptomatic tendon (i.e., contralateral tendon) was used as the denominator (i.e., comparator) to compute the asymmetry index may have attenuated the magnitude of asymmetry, as both tendons may be structurally compromised in individuals with unilateral AT (30). Hence, the inclusion of healthy matched controls could have enriched the findings (81). Finally, the addition of MUBs expressing neovascularization (i.e., Doppler) or stiffness (i.e., elastography) of the tendon could have strengthened the results of this study (85).
Conclusion
Musculoskeletal ultrasound biomarkers have a clinical utility in visualizing in vivo tendon integrity. They add valuable diagnostic information when midportion AT is suspected in clinical practice and research protocols. However, MUBs among individuals with chronic AT are at best weakly associated with pain, flexibility, strength, and functional capacity. In that sense, MUBs are to be valued as part of a multimodal assessment, as they complement patient-reported outcome measures targeting pain and activity limitations and clinically based measures such as ankle flexibility and strength. These last two types of measures may provide greater insight into the symptomatology and functional impacts of chronic AT than MUBs alone and best inform the rehabilitation treatment plan.
Data Availability Statement
The raw data supporting the conclusions of this article will be made available by the authors, without undue reservation.
Ethics Statement
The studies involving human participants were reviewed and approved by Centre for Interdisciplinary Research in Rehabilitation of Greater Montreal (CRIR) Research Ethics Committee. The patients/participants provided their written informed consent to participate in this study.
Author Contributions
MLal and DG contributed to the design of the project, development of the methodology, research ethics approval process, collection of data, validation, analysis and interpretation, writing of the original draft of the manuscript, final review and editing of the manuscript, project administration, and acquisition of funding. SP and M-JN contributed to the acquisition of data, validation and analysis, the writing of the original draft of the manuscript, and the final review and editing of the manuscript. CL, MLam, and FD contributed to the design of the project, development of the methodology, data analysis and interpretation, the final review and editing of the manuscript, and acquisition of funding. All authors contributed to the article and approved the submitted version.
Funding
The project was funded through a Clinical Research Partnership in Physiotherapy supported by the Ordre Professionnel de la Physiothérapie du Québec and the Quebec Rehabilitation Research Network. MLal holds a doctoral training scholarship from the Fonds de la recherche en santé du Québec (FRSQ). FD and DG hold a Research Career Award from the FRSQ and DG co-chairs the Initiative for the Development of New Technologies and Practices in Rehabilitation (INSPIRE) funded by the LRH Foundation. The equipment and material needed for work completed at the Pathokinesiology Laboratory were funded in part by the Canada Foundation for Innovation (CFI).
Conflict of Interest
The authors declare that the research was conducted in the absence of any commercial or financial relationships that could be construed as a potential conflict of interest.
Publisher's Note
All claims expressed in this article are solely those of the authors and do not necessarily represent those of their affiliated organizations, or those of the publisher, the editors and the reviewers. Any product that may be evaluated in this article, or claim that may be made by its manufacturer, is not guaranteed or endorsed by the publisher.
Acknowledgments
Special thanks are extended to Dr. Amélie Desroches who assisted with data collection and analysis.
References
1. de Jonge S, Van den Berg C, de Vos R-J, Van Der Heide H, Weir A, Verhaar J, et al. Incidence of midportion Achilles tendinopathy in the general population. Br J Sports Med. (2011) 45:1026–8. doi: 10.1136/bjsports-2011-090342
2. Alfredson H, Lorentzon R. Chronic achilles tendinosis. Sports Med. (2000) 29:135–46. doi: 10.2165/00007256-200029020-00005
3. Rolf C, Movin T. Etiology, histopathology, and outcome of surgery in achillodynia. Foot Ankle Int. (1997) 18:565–9. doi: 10.1177/107110079701800906
4. Martin RL, Chimenti R, Cuddeford T, Houck J, Matheson J, McDonough CM, et al. Achilles pain, stiffness, and muscle power deficits: midportion achilles tendinopathy revision 2018: clinical practice guidelines linked to the international classification of functioning, disability and health from the orthopaedic section of the American Physical Therapy Association. J Orthop Sports Phys Ther. (2018) 48:A1–38. doi: 10.2519/jospt.2018.0302
5. Docking SI, Cook J. How do tendons adapt? Going beyond tissue responses to understand positive adaptation and pathology development: A narrative review. J Musculoskelet Neuronal Interact. (2019) 19:300–10.
6. Scott A, Backman LJ, Speed C. Tendinopathy: update on pathophysiology. J Orthop Sports Phys Ther. (2015) 45:833–41. doi: 10.2519/jospt.2015.5884
7. van der Vlist AC, Breda SJ, Oei EHG, Verhaar JAN, de Vos R-J. Clinical risk factors for Achilles tendinopathy: a systematic review. Br J Sports Med. (2019) 53:1352–61. doi: 10.1136/bjsports-2018-099991
8. Rabin A, Kozol Z, Finestone AS. Limited ankle dorsiflexion increases the risk for mid-portion Achilles tendinopathy in infantry recruits: a prospective cohort study. J Foot Ankle Res. (2014) 7:48. doi: 10.1186/s13047-014-0048-3
9. O'Neill S, Barry S, Watson P. Plantarflexor strength and endurance deficits associated with mid-portion Achilles tendinopathy: the role of soleus. Physical Therapy in Sport. (2019) 37:69–76. doi: 10.1016/j.ptsp.2019.03.002
10. Järvinen M, Jozsa L, Kannus P, Järvinen T, Kvist M, Leadbetter W. Histopathological findings in chronic tendon disorders. Scand J Med Sci Sports. (1997) 7:86–95. doi: 10.1111/j.1600-0838.1997.tb00124.x
11. Maffulli N. Overuse tendon conditions: time to change a confusing terminology. Arthroscopy. (1998) 14:840–3. doi: 10.1016/S0749-8063(98)70021-0
12. Kulig K, Chang Y-J, Winiarski S, Bashford GR. Ultrasound-based tendon micromorphology predicts mechanical characteristics of degenerated tendons. Ultrasound Med Biol. (2016) 42:664–73. doi: 10.1016/j.ultrasmedbio.2015.11.013
13. Cook J, Purdam CR. Is tendon pathology a continuum? A pathology model to explain the clinical presentation of load-induced tendinopathy. Br J Sports Med. (2009) 43:409–16. doi: 10.1136/bjsm.2008.051193
14. Obst SJ, Heales LJ, Schrader BL, Davis SA, Dodd KA, Holzberger CJ, et al. Are the mechanical or material properties of the achilles and patellar tendons altered in tendinopathy? A Systematic Review with Meta-analysis. Sports Med. (2018) 48:2179–98. doi: 10.1007/s40279-018-0956-7
15. Lalumiere M, Larivière C, Nadeau MJ, Paquette P, Lamontagne M, Desmeules F, et al. Proposing a minimal data set of musculoskeletal ultrasound imaging biomarkers to inform clinical practice: an analysis founded on the achilles tendon. Ultrasound Med Biol. (2020) 46:2222–35. doi: 10.1016/j.ultrasmedbio.2020.04.024
16. Ooi C, Schneider M, Malliaras P, Chadwick M, Connell D. Diagnostic performance of sonoelastography in confirming clinically diagnosed achilles tendinopathy: Comparison with B-mode ultrasound and color doppler imaging. European Congress of Radiology-2014 CSM (2014). doi: 10.1594/ranzcr2014/R-0273
17. Sunding K, Fahlström M, Werner S, Forssblad M, Willberg L. Evaluation of Achilles and patellar tendinopathy with greyscale ultrasound and colour Doppler: using a four-grade scale. Knee Surg Sports Traumatol Arthrosc. (2014) 24:1988–96. doi: 10.1007/s00167-014-3270-4
18. Van Schie H, de Vos R-J, de Jonge S, Bakker E, Heijboer M, Verhaar J, et al. Ultrasonographic tissue characterisation of human Achilles tendons: quantification of tendon structure through a novel non-invasive approach. Br J Sports Med. (2010) 44:1153–9. doi: 10.1136/bjsm.2009.061010
19. Nadeau M-J, Desrochers A, Lamontagne M, Larivière C, Gagnon DH. Quantitative ultrasound imaging of Achilles tendon integrity in symptomatic and asymptomatic individuals: reliability and minimal detectable change. J Foot Ankle Res. (2016) 9:30. doi: 10.1186/s13047-016-0164-3
20. Matthews W, Ellis R, Furness JW, Rathbone E, Hing W. Staging achilles tendinopathy using ultrasound imaging: the development and investigation of a new ultrasound imaging criteria based on the continuum model of tendon pathology. BMJ Open Sport Exerc Med. (2020) 6:e000699. doi: 10.1136/bmjsem-2019-000699
21. Alfredson H, Pietilä T, Jonsson P, Lorentzon R. Heavy-load eccentric calf muscle training for the treatment of chronic Achilles tendinosis. Am J Sports Med. (1998) 26:360–6. doi: 10.1177/03635465980260030301
22. Åström M, Gentz C-F, Nilsson P, Rausing A, Sjöberg S, Westlin N. Imaging in chronic Achilles tendinopathy: a comparison of ultrasonography, magnetic resonance imaging and surgical findings in 27 histologically verified cases. Skeletal Radiol. (1996) 25:615–20. doi: 10.1007/s002560050146
23. Abat F, Maffulli N, Alfredson H, Lopez-Vidriero E, Myers C, Gomes S, et al. Clinical Utility of Diagnostic Ultrasound in Athletes with Tendinopathy (ICL 22). ESSKA Instructional Course Lecture Book. Barcelona: Springer (2016). p. 217–23. doi: 10.1007/978-3-662-49114-0_19
24. Sussmilch-Leitch SP, Collins NJ, Bialocerkowski AE, Warden SJ, Crossley KM. Physical therapies for Achilles tendinopathy: systematic review and meta-analysis. J Foot Ankle Res. (2012) 5:1. doi: 10.1186/1757-1146-5-15
25. Bakkegaard M, Johannsen FE, Højgaard B, Langberg H. Ultrasonography as a prognostic and objective parameter in Achilles tendinopathy: a prospective observational study. Eur J Radiol. (2015) 84:458–62. doi: 10.1016/j.ejrad.2014.11.028
26. de Vos RJ, Heijboer MP, Weinans H, Verhaar JA, van Schie HT. Tendon structure's lack of relation to clinical outcome after eccentric exercises in chronic midportion Achilles tendinopathy. J Sport Rehabil. (2012) 21:34–43. doi: 10.1123/jsr.21.1.34
27. Mkumbuzi NS, Jørgensen OH, Mafu TS, September AV, Posthumus M, Collins M. Ultrasound findings are not associated with tendon pain in recreational athletes with chronic Achilles tendinopathy. Transl Sports Med. (2020) 3:589–98. doi: 10.1002/tsm2.183
28. de Jonge S, Tol JL, Weir A, Waarsing JH, Verhaar JA, de Vos RJ. The tendon structure returns to asymptomatic values in non-operatively treated achilles tendinopathy but is not associated with symptoms: a prospective study. Am J Sports Med. (2015) 43:2950–8. doi: 10.1177/0363546515605077
29. Docking SI, Rio E, Cook J, Carey D, Fortington L. Quantification of Achilles and patellar tendon structure on imaging does not enhance ability to predict self-reported symptoms beyond grey-scale ultrasound and previous history. J Sci Med Sport. (2018) 22:145–50. doi: 10.1016/j.jsams.2018.07.016
30. Docking SI, Rosengarten SD, Daffy J, Cook J. Structural integrity is decreased in both Achilles tendons in people with unilateral Achilles tendinopathy. J Sci Med Sport. (2015) 18:383–7. doi: 10.1016/j.jsams.2014.06.004
31. Emerson C, Morrissey D, Perry M, Jalan R. Ultrasonographically detected changes in Achilles tendons and self reported symptoms in elite gymnasts compared with controls–an observational study. Man Ther. (2010) 15:37–42. doi: 10.1016/j.math.2009.05.008
32. Stecco A, Busoni F, Stecco C, Mattioli-Belmonte M, Soldani P, Condino S, et al. Comparative ultrasonographic evaluation of the Achilles paratenon in symptomatic and asymptomatic subjects: an imaging study. Surg Radiol Anat. (2015) 37:281–5. doi: 10.1007/s00276-014-1338-y
33. van Ark M, Rio E, Cook J, van den Akker-Scheek I, Gaida JE, Zwerver J, et al. Clinical improvements are not explained by changes in tendon structure on ultrasound tissue characterization after an exercise program for patellar tendinopathy. Am J Phys Med Rehabil. (2018) 97:708–14. doi: 10.1097/PHM.0000000000000951
34. van der Plas A, de Jonge S, de Vos RJ, van der Heide HJ, Verhaar JA, Weir A, et al. A 5-year follow-up study of Alfredson's heel-drop exercise programme in chronic midportion Achilles tendinopathy. Br J Sports Med. (2012) 46:214–8. doi: 10.1136/bjsports-2011-090035
35. Corrigan P, Cortes DH, Pohlig RT, Grävare Silbernagel K. Tendon morphology and mechanical properties are associated with the recovery of symptoms and function in patients with Achilles tendinopathy. Orthop J Sports Med. (2020) 8:2325967120917271. doi: 10.1177/2325967120917271
36. Beyer R, Kongsgaard M, Kjær BH, Øhlenschlæger T, Kjær M, Magnusson SP. Heavy slow resistance versus eccentric training as treatment for achilles tendinopathy a randomized controlled trial. Am J Sports Med. (2015) 43:1704–11. doi: 10.1177/0363546515584760
37. Öhberg L, Lorentzon R, Alfredson H. Eccentric training in patients with chronic Achilles tendinosis: normalised tendon structure and decreased thickness at follow up. Br J Sports Med. (2004) 38:8–11. doi: 10.1136/bjsm.2001.000284
38. Vestergrd Iversen J, Bartels EM, Langberg H. The Victorian Institute of Sports Assessment-Achilles questionnaire (VISA-A)-reliable tool for measuring achilles tendinopathy. Int J Sports Phys Ther. (2012) 7:76–84.
39. Robinson J, Cook JL, Purdam C, Visentini P, Ross J, Maffulli N, et al. The VISA-A questionnaire: a valid and reliable index of the clinical severity of Achilles tendinopathy. Br J Sports Med. (2001) 35:335–41. doi: 10.1136/bjsm.35.5.335
40. Kaux J-F, Delvaux F, Oppong-Kyei J, Dardenne N, Beaudart C, Buckinx F, et al. Validity and reliability of the French translation of the VISA-A questionnaire for Achilles tendinopathy. Disabil Rehabil. (2016) 38:2593–9. doi: 10.3109/09638288.2016.1138553
41. McCormack J, Underwood F, Slaven E, Cappaert T. The minimum clinically important difference on the VISA-A and LEFS for patients with insertional achilles tendinopathy. Int J Sports Phys Ther. (2015) 10:639.
42. Binkley JM, Stratford PW, Lott SA, Riddle DL. The Lower Extremity Functional Scale (LEFS): scale development, measurement properties, and clinical application. Phys Ther. (1999) 79:371–83. doi: 10.1037/t35109-000
43. Powden CJ, Hoch JM, Hoch MC. Reliability and minimal detectable change of the weight-bearing lunge test: a systematic review. Man Ther. (2015) 20:524–32. doi: 10.1016/j.math.2015.01.004
45. Milot M-H, Nadeau S, Gravel D. Muscular utilization of the plantarflexors, hip flexors and extensors in persons with hemiparesis walking at self-selected and maximal speeds. J Electromyogr Kinesiol. (2007) 17:184–93. doi: 10.1016/j.jelekin.2006.01.001
46. Papaiordanidou M, Mustacchi V, Stevenot JD, Vanoncini M, Martin A. Spinal and supraspinal mechanisms affecting torque development at different joint angles. Muscle Nerve. (2016) 53:626–32. doi: 10.1002/mus.24895
47. Don R, Ranavolo A, Cacchio A, Serrao M, Costabile F, Iachelli M, et al. Relationship between recovery of calf-muscle biomechanical properties and gait pattern following surgery for Achilles tendon rupture. Clin Biomech. (2007) 22:211–20. doi: 10.1016/j.clinbiomech.2006.10.001
48. Arya S, Kulig K. Tendinopathy alters mechanical and material properties of the Achilles tendon. J Appl Physiol. (2010) 108:670–5. doi: 10.1152/japplphysiol.00259.2009
49. Petrovic M, Maganaris CN, Deschamps K, Verschueren SM, Bowling FL, Boulton AJM, et al. Altered Achilles tendon function during walking in people with diabetic neuropathy: implications for metabolic energy saving. J Appl Physiol. (2018) 124:1333–40. doi: 10.1152/japplphysiol.00290.2017
50. Holmbäck AM, Porter MM, Downham D, Lexell J. Reliability of isokinetic ankle dorsiflexor strength measurements in healthy young men and women. Scand J Rehabil Med. (1999) 31:229–39. doi: 10.1080/003655099444407
51. Taylor NA, Sanders RH, Howick EI, Stanley SN. Static and dynamic assessment of the Biodex dynamometer. Eur J Appl Physiol Occup Physiol. (1991) 62:180–8. doi: 10.1007/BF00643739
52. Feiring DC, Ellenbecker TS, Derscheid GL. Test-retest reliability of the Biodex isokinetic dynamometer. J Orthop Sports Phys Ther. (1990) 11:298–300. doi: 10.2519/jospt.1990.11.7.298
53. Schneebeli A, Del Grande F, Vincenzo G, Cescon C, Barbero M. Test-retest reliability of echo intensity parameters in healthy Achilles tendons using a semi-automatic tracing procedure. Skeletal Radiol. (2017) 46:1553–8. doi: 10.1007/s00256-017-2748-9
54. Collinger JL, Gagnon D, Jacobson J, Impink BG, Boninger ML. Reliability of quantitative ultrasound measures of the biceps and supraspinatus tendons. Acad Radiol. (2009) 16:1424–32. doi: 10.1016/j.acra.2009.05.001
55. Hedges LV. Distribution theory for glass's estimator of effect size and related estimators. J Educ Stat. (1981) 6:107–28. doi: 10.3102/10769986006002107
56. Sawilowsky SS. New effect size rules of thumb. J Mod Appl Stat Methods. (2009) 8:26. doi: 10.22237/jmasm/1257035100
57. Mukaka MM. A guide to appropriate use of correlation coefficient in medical research. Malawi Med J. (2012) 24:69–71.
58. Cook J, Rio E, Purdam C, Docking S. Revisiting the continuum model of tendon pathology: what is its merit in clinical practice and research? Br J Sports Med. (2016) 50:1–7. doi: 10.1136/bjsports-2015-095422
59. Cook JL, Feller JA, Bonar SF, Khan KM. Abnormal tenocyte morphology is more prevalent than collagen disruption in asymptomatic athletes' patellar tendons. J Orthop Res. (2004) 22:334–8. doi: 10.1016/j.orthres.2003.08.005
60. Docking SI, Ooi CC, Connell D. Tendinopathy: is imaging telling us the entire story? J Orthop Sports Phys Ther. (2015) 45:842–52. doi: 10.2519/jospt.2015.5880
61. Sánchez Romero EA, Pollet J, Martín Pérez S, Alonso Pérez JL, Muñoz Fernández AC, Pedersini P, et al. Lower limb tendinopathy tissue changes assessed through ultrasound: a narrative review. Medicina. (2020) 56:378. doi: 10.3390/medicina56080378
62. Matthews W, Ellis R, Furness J, Hing W. Classification of tendon matrix change using ultrasound imaging: a systematic review and meta-analysis. Ultrasound Med Biol. (2018) 44:2059–80. doi: 10.1016/j.ultrasmedbio.2018.05.022
63. Romero-Morales C, Martín-Llantino PJ, Calvo-Lobo C, Palomo-López P, López-López D, Pareja-Galeano H, et al. Comparison of the sonographic features of the Achilles Tendon complex in patients with and without achilles tendinopathy: a case-control study. Phys Ther Sport. (2019) 35:122–6. doi: 10.1016/j.ptsp.2018.12.003
64. McAuliffe S, McCreesh K, Culloty F, Purtill H, O'Sullivan K. Can ultrasound imaging predict the development of Achilles and patellar tendinopathy? A systematic review and meta-analysis. Br J Sports Med. (2016) 50:1516–23. doi: 10.1136/bjsports-2016-096288
65. Liu S, Wang Y, Yang X, Lei B, Liu L, Li SX, et al. Deep learning in medical ultrasound analysis: a review. Engineering. (2019) 5:261–75. doi: 10.1016/j.eng.2018.11.020
66. Rio E, Moseley L, Purdam C, Samiric T, Kidgell D, Pearce AJ, et al. The pain of tendinopathy: physiological or pathophysiological? Sports Med. (2014) 44:9–23. doi: 10.1007/s40279-013-0096-z
67. Docking SI, Girdwood MA, Cook J, Fortington LV, Rio E. Reduced levels of aligned fibrillar structure are not associated with achilles and patellar tendon symptoms. Clin J Sport Med. (2020) 30:550–5. doi: 10.1097/JSM.0000000000000644
68. Agergaard A-S, Svensson RB, Malmgaard-Clausen NM, Couppé C, Hjortshoej MH, Doessing S, et al. Clinical outcomes, structure, and function improve with both heavy and moderate loads in the treatment of patellar tendinopathy: a randomized clinical trial. Am J Sports Med. (2021) 49:982–93. doi: 10.1177/0363546520988741
69. Järvinen TA. Neovascularisation in tendinopathy: from eradication to stabilisation? Br J Sports Med. (2020) 54:1–2. doi: 10.1136/bjsports-2019-100608
70. Christensen J, Alfredson H, Andersson G. Protease-activated receptors in the Achilles tendon–a potential explanation for the excessive pain signalling in tendinopathy. Mol Pain. (2015) 11:13. doi: 10.1186/s12990-015-0007-4
71. Gouveia-Figueira S, Nording ML, Gaida JE, Forsgren S, Alfredson H, Fowler CJ. Serum levels of oxylipins in achilles tendinopathy: an exploratory study. PLoS ONE. (2015) 10:e0123114. doi: 10.1371/journal.pone.0123114
72. Mascarenhas S. A narrative review of the classification and use of diagnostic ultrasound for conditions of the achilles tendon. Diagnostics. (2020) 10:944. doi: 10.3390/diagnostics10110944
73. Pelletier R, Higgins J, Bourbonnais D. Is neuroplasticity in the central nervous system the missing link to our understanding of chronic musculoskeletal disorders? BMC Musculoskelet Disord. (2015) 16:25. doi: 10.1186/s12891-015-0480-y
74. Tompra N, van Dieën JH, Coppieters MW. Central pain processing is altered in people with Achilles tendinopathy. Br J Sports Med. (2016) 50:1004–7. doi: 10.1136/bjsports-2015-095476
75. Silbernagel KG, Hanlon S, Sprague A. Current clinical concepts: conservative management of Achilles tendinopathy. J Athl Train. (2020) 55:438–47. doi: 10.4085/1062-6050-356-19
76. Corrigan P, Cortes DH, Pontiggia L, Silbernagel KG. The degree of tendinosis is related to symptom severity and physical activity levels in patients with midportion Achilles tendinopathy. Int J Sports Phys Ther. (2018) 13:196–207. doi: 10.26603/ijspt20180196
77. French DJ, France CR, Vigneau F, French JA, Evans RT. Fear of movement/(re)injury in chronic pain: a psychometric assessment of the original English version of the Tampa scale for kinesiophobia (TSK). Pain. (2007) 127:42–51. doi: 10.1016/j.pain.2006.07.016
78. Khullar S, Gamage P, Malliaras P, Huguenin L, Prakash A, Connell D. Prevalence of coexistent plantaris tendon pathology in patients with mid-portion achilles pathology: a retrospective MRI study. Sports. (2019) 7:124. doi: 10.3390/sports7050124
79. Spang C, Harandi V, Alfredson H, Forsgren S. Marked innervation but also signs of nerve degeneration in between the Achilles and plantaris tendons and presence of innervation within the plantaris tendon in midportion Achilles tendinopathy. J Musculoskelet Neuronal Interact. (2015) 15:197–206.
80. McAuliffe S, Tabuena A, McCreesh K, O'Keeffe M, Hurley J, Comyns T, et al. Altered strength profile in achilles tendinopathy: a systematic review and meta-Analysis. J Athl Train. (2019) 54:889–900. doi: 10.4085/1062-6050-43-18
81. Heales LJ, Lim ECW, Hodges PW, Vicenzino B. Sensory and motor deficits exist on the non-injured side of patients with unilateral tendon pain and disability—implications for central nervous system involvement: a systematic review with meta-analysis. Br J Sports Med. (2014) 48:1400–6. doi: 10.1136/bjsports-2013-092535
82. Andersson G, Forsgren S, Scott A, Gaida JE, Stjernfeldt JE, Lorentzon R, et al. Tenocyte hypercellularity and vascular proliferation in a rabbit model of tendinopathy: contralateral effects suggest the involvement of central neuronal mechanisms. Br J Sports Med. (2011) 45:399–406. doi: 10.1136/bjsm.2009.068122
83. Azevedo LB, Lambert MI, Vaughan CL, O'Connor CM, Schwellnus MP. Biomechanical variables associated with achilles tendinopathy in runners. Br J Sports Med. (2008) 43:288–92. doi: 10.1136/bjsm.2008.053421
84. Masci L, Spang C, van Schie HT, Alfredson H. How to diagnose plantaris tendon involvement in midportion Achilles tendinopathy - clinical and imaging findings. BMC Musculoskelet Disord. (2016) 17:97. doi: 10.1186/s12891-016-0955-5
85. Gatz M, Betsch M, Bode D, Schweda S, Dirrichs T, Migliorini F, et al. Intra individual comparison of unilateral Achilles tendinopathy using B-mode, power Doppler, ultrasound tissue characterization and shear wave elastography. J Sports Med Phys Fitness. (2020) 60:1462–9. doi: 10.23736/S0022-4707.20.11031-4
Keywords: computer-assisted image analysis, diagnostic imaging, image processing, musculoskeletal – disorders, rehabilitation, tendinosis, tendinopathy, ultrasonography
Citation: Lalumiere M, Perrino S, Nadeau M-J, Larivière C, Lamontagne M, Desmeules F and H. Gagnon D (2021) To What Extent Do Musculoskeletal Ultrasound Biomarkers Relate to Pain, Flexibility, Strength, and Function in Individuals With Chronic Symptomatic Achilles Tendinopathy? Front. Rehabilit. Sci. 2:726313. doi: 10.3389/fresc.2021.726313
Received: 16 June 2021; Accepted: 08 July 2021;
Published: 12 August 2021.
Edited by:
Chueh-Hung Wu, National Taiwan University Hospital, TaiwanReviewed by:
Ming-Yen Hsiao, National Taiwan University, TaiwanKamal Mezian, Charles University, Czechia
Copyright © 2021 Lalumiere, Perrino, Nadeau, Larivière, Lamontagne, Desmeules and H. Gagnon. This is an open-access article distributed under the terms of the Creative Commons Attribution License (CC BY). The use, distribution or reproduction in other forums is permitted, provided the original author(s) and the copyright owner(s) are credited and that the original publication in this journal is cited, in accordance with accepted academic practice. No use, distribution or reproduction is permitted which does not comply with these terms.
*Correspondence: Dany H. Gagnon, dany.gagnon.2@umontreal.ca orcid.org/0000-0003-3464-4667