A Developmental Framework for Embodiment Research: The Next Step Toward Integrating Concepts and Methods
- 1Department of Genetic Psychology, Faculty of Psychology, Ruhr-Universität Bochum, Bochum, Germany
- 2Department of Anthropology, University of California, San Diego, La Jolla, CA, United States
- 3Department of Psychology, University of Calgary, Calgary, AB, Canada
- 4Chair of Human Movement Science, Department of Sports and Health Sciences, Technical University of Munich, Munich, Germany
- 5Department of Psychiatry, Oxford Centre for Human Brain Activity, Warneford Hospital, Oxford, United Kingdom
- 6Translational Neuromodeling Unit, Institute for Biomedical Engineering, University of Zurich and ETH Zurich, Zurich, Switzerland
- 7Institute of Sports Science, Faculty of Humanities, Leibniz University Hannover, Hannover, Germany
Embodiment research is at a turning point. There is an increasing amount of data and studies investigating embodiment phenomena and their role in mental processing and functions from across a wide range of disciplines and theoretical schools within the life sciences. However, the integration of behavioral data with data from different biological levels is challenging for the involved research fields such as movement psychology, social and developmental neuroscience, computational psychosomatics, social and behavioral epigenetics, human-centered robotics, and many more. This highlights the need for an interdisciplinary framework of embodiment research. In addition, there is a growing need for a cross-disciplinary consensus on level-specific criteria of embodiment. We propose that a developmental perspective on embodiment is able to provide a framework for overcoming such pressing issues, providing analytical tools to link timescales and levels of embodiment specific to the function under study, uncovering the underlying developmental processes, clarifying level-specific embodiment criteria, and providing a matrix and platform to bridge disciplinary boundaries among the involved research fields.
Introduction
Embodiment has become a key concept in human life sciences in recent years. Although generally understood as the sum of bodily preconditions of cognition, emotion, and behavior, a closer look unveils that conceptualizations of embodiment vary strongly among research areas and theoretical schools: from embodied simulation to embodied cognition, from biological embedding of experience to interoception and the embodied mind. Differences also include types of knowledge production (e.g., cognitive processing models vs. motor developmental pathways vs. computational simulations of neural activity patterns), data modalities (e.g., self-reports vs. medical diagnoses vs. movement trajectories vs. molecular epigenetic profiles), and the involved levels of analysis (e.g., psychological function vs. gene expression). Different research areas and theoretical schools also account for different timescales across which embodiment processes can be analyzed (e.g., life-time effects vs. acute activation). Importantly, some of the most pressing topics with the greatest potential to advance embodiment research cut across research disciplines and are currently significantly hampered by the lack of a common theoretical framework that bridges the different conceptualizations of embodiment.
The current situation calls for an interdisciplinary conceptualization of embodiment. Here, we propose to adopt a developmental perspective as an integrative cross-disciplinary framework of embodiment research serving three main purposes: A developmental perspective on embodiment accounts for the different timescales that underlie the processes of incorporation and expression of an agent's embodied experiences in the interaction with the environment. It also relates different levels of embodiment to one another as they develop over the lifespan, based on their biological and functional interconnectedness. Finally, it clarifies disciplinary boundaries and finds connection points by identifying transmission hubs between the levels involved in a particular embodiment process which, then, creates links for collaboration between participating disciplines. This is especially important for research areas which try to overcome disciplinary limits but are still bound to the methods and committed to research standards within their respective field. Thus, on the basis of those three purposes, the proposed framework does not aim at providing a new definition of embodiment but shall be understood as a platform and analytical tool to enable and support integrative cross-disciplinary embodiment research by clarifying critical aspects that currently hamper the advance in the field.
In the following, we will, first, characterize two main types of approaches to embodiment in the life sciences, agency and environmental approaches (see section Integrating Environmental and Agency Approaches to Embodiment) and discuss how they conceptualize developmental processes underlying embodiment phenomena (see section Developmental Processes Grounding Embodiment: Implicit and Underexplored). Due to the variety of embodiment research in the life sciences, these two sections do not cover all existing embodiment concepts. We focus on those fields of embodiment research and concepts of embodiment for which adapting an integrative developmental perspective would be most productive from our perspective. Second, we will present examples for the potential and the challenges of developmental embodiment research (see section Developmental Embodiment Research: Cross-Disciplinary Examples). Finally, we discuss our framework of developmental embodiment research in more detail (see section Toward an Integrative Framework for Developmental Embodiment Research).
Integrating Environmental and Agency Approaches to Embodiment
Developmental embodiment research, as we propose it in this paper, builds on two distinct lines of current embodiment research in the life sciences, which rarely connect conceptually, or in empirical studies (see Box 1 and Table 1 for an introduction into key concepts, targeted embodiment phenomena and related key references): First, “agency approaches” that emphasize how bodily embeddedness, anatomic preconditions, and physiological as well as neurophysiological foundations momentarily enable movements, actions, and psychological functions. Second, “environmental approaches,” which describe how the physical, social, and cultural environment is incorporated, and affects the physical structure of the body or brain and subsequently its function. Agency approaches mainly focus on the acute activation and involvement of the body, including basal body functions, signals, resources, and conditions etc. with emphasis on the active interaction with the environment. Research originating from an agency approach investigates how embodied experiences are mobilized and how they influence interactions with the environment. Environmental approaches, in contrast, focus on the long-term impact of environmental signals on bodily preconditions of mental functions and mental health outcomes. Research coming from an environmental approach investigates how environmental (pre)conditions and events are incorporated into the body to become embodied experiences. With our developmental framework, we propose to integrate agency and environmental approaches by understanding the body as a reservoir of experiences, providing a sort of storage and ‘memory' of experiences and capabilities necessary for action and mental functions which are activated and mobilized in the specific moment of interaction with the environment.
Box 1. Approaches to embodiment in the life sciences which contribute to the proposed developmental perspective.
The rise of embodiment concepts in the life sciences started out in the 1980s, when linguists, cognitive psychologist, and robotic engineers turned to the study of motor and sensory processing, emphasizing that they shape the way our mind works. Neuroscience and philosophy of mind contributed to further advance this perspective (Varela et al., 1991; Gibbs, 2005; Shapiro, 2011). Subsequently, a range of different concepts developed by building up on the joint idea that our bodily preconditions are the foundation for the perception of ourselves (in the sense of consciousness and interoception) and the perception of the environment with which we interact, affecting a range of processes and phenomena related to e.g., cognition, emotion processing, social interaction, aesthetic perception, and mental health. We subsume these as agency approaches to embodiment (see Table 1).
While the lines along which different embodiment concepts should be distinguished are still highly debated (for embodied cognition, being the most prominent concept of an agency approach to embodiment, see e.g., Clark, 1999; Wilson, 2002; Overton et al., 2008; Kiverstein, 2012; Meteyard et al., 2012), they were picked up quickly in cognitive psychology and other related fields of research (see Table 1). Here, they provided a platform for the use of the newly established neurofunctional approach based on neuroimaging techniques (mainly fMRI) within the existing cognitive paradigm. In sports psychology and movement science, for example, the concepts of embodied cognition and embodied simulation bridged the gap between neurocognitive research and practical knowledge based on motor expertise, movement learning, as well as motor rehabilitation techniques. Further, the acknowledgment of the embeddedness of our mind within our most basal bodily functions via interoceptive pathways (see Craig, 2004, 2009a,b) and their influence on emotion processing, self-awareness, and time perception, strongly influenced research fields such as computational psychiatry and psychosomatics, and mental health research (Petzschner et al., 2017; Khalsa et al., 2018). Here, we already observe first steps to integrate agency approaches to embodiment with those studying processes of incorporation of experiences at several underlying biological levels (Petzschner et al., 2017).
This turn to the bodily preconditions of action, perception, and emotions met with a turn to the body in population health and epidemiological research (see Krieger, 2005; Hertzman, 2012; Rutter, 2012; Gluckman et al., 2016). The question was whether and how adverse or advantageous environmental conditions, experienced during sensitive periods in life (e.g., pre- or perinatal), become incorporated into the body and subsequently constitute divergent developmental pathways of physical and mental health. The concepts used to describe such long-term effects of environmental impacts, which we subsume as environmental approaches to embodiment (see Table 1), focus on different physiological levels of the body underlying mental functions, e.g., (psycho)physiological feedback mechanisms, hormone regulation, neural networks, brain anatomy, inflammatory processes, gene-environment interactions, as well as epigenetic mechanisms (e.g., Godfrey et al., 2007; Wadhwa et al., 2009; Danese et al., 2011; Rutter, 2012; Nelson, 2017; Bush et al., 2018; Aristizabal et al., 2019). We limit our discussion here to those studying the impact on mental health. While these concepts differ in their focus on the time-point and duration of the environmental impact, spanning transient to persistent effects, they all attribute a central role to developmental processes in the translation of the environmental impact to later mental health outcome. Thus, the classification of developmental pathways underlying diverse outcomes is a joint important goal in these concepts. It is also assumed necessary for translational research and future intervention (Rutter, 2016).
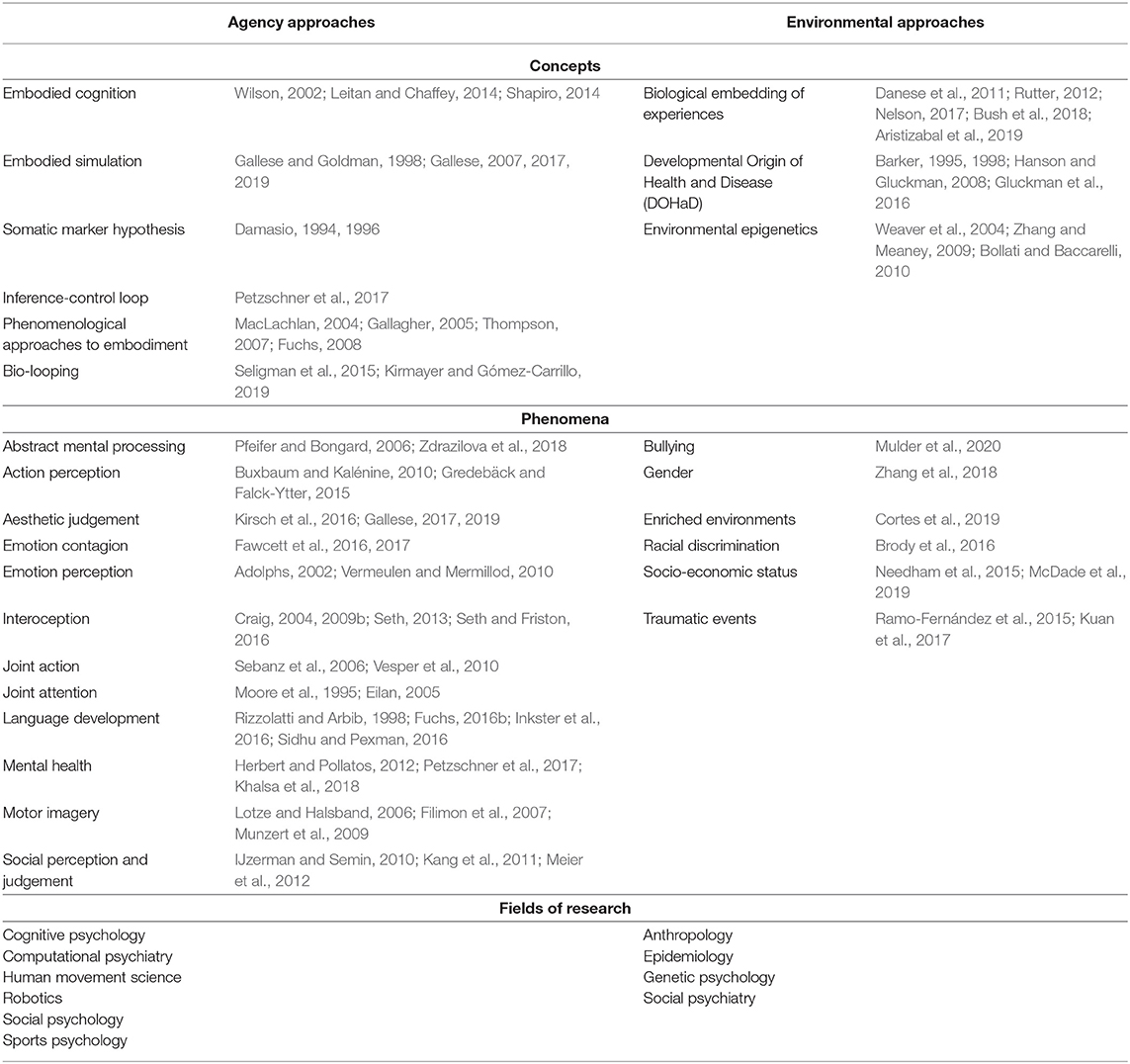
Table 1. Examples of agency and environmental approaches to embodiment: concepts, phenomena and fields of research.
Other attempts to classify embodiment approaches often distinguish a third group of phenomenological approaches (Overton, 2008), or further differentiate along methods (experiential/objective), epistemological perspective (1st, 2nd, and 3rd third person perspective), and object of research (cognitive structure/practice) (Hornecker et al., 2017). We subsume phenomenological approaches under the group of agency approaches, as these are also primarily concerned with the momentary preconditions of consciousness, action, or perception, although from a first (or second) person perspective.
Integrating agency and environmental approaches to embodiment clearly broadens the notion of embodiment compared to its use in discipline-specific research areas (e.g., in embodied cognition research, see Wilson, 2002). In addition to different neural networks involved, e.g., in decision making, abstract word recognition, and movement execution, embodiment processes also encompass the physical constitution of the body (the anatomic structure of the limbs, homeostatic feedback processes, hormonal balance, basic sensory organization, and function, etc.), the neural networks involved in the regulation of these processes, and the molecular underpinnings of these regulation processes and network constitutions. This broader notion of embodiment further includes the focus on the anatomic structure of the body used in robotics (see Pfeifer and Bongard, 2006), the notion of an extended mind to our immediate environment and, most importantly, intersubjectivity emphasized in phenomenological accounts (see Fuchs, 2017), as well as the biological embedding of experiences across the lifespan (see Rutter, 2012). Still, not all body processes and conditions are of interest for developmental embodiment research, but only those which participate in the incorporation, shaping, and expression of embodiment experiences, in the context of e.g., a particular mental function or mental health outcome under study. Developmental embodiment research, as we propose it here, provides a matrix and platform through which different approaches of embodiment research can collaborate in the interdisciplinary study of a particular mental function or mental health outcome.
Developmental Processes Grounding Embodiment: Implicit and Underexplored
Although developmental theories are one of the historical pillars of embodiment research (Overton and Lerner, 2012), the question of how embodied functions develop often comes second in empirical studies. Some authors even question that a developmental perspective contributes at all to elucidate the underlying mechanisms of embodiment phenomena (e.g., Körner et al., 2015). In our view, both the agency approaches and the environmental approaches to embodiment imply developmental processes and would therefore profit from an explicit developmental perspective.
Agency approaches, on the one hand, implicitly acknowledge lifespan developmental changes in e.g., cognitive and motor functioning within their theoretical framework, but the specific impact on the embodiment phenomena under study is not empirically investigated. Referencing Gibson's ecological theory (Gibson, 1986//2014) or Piaget's stages of cognitive development (Inhelder and Piaget, 1958; Piaget, 1977), for example, agency approaches emphasize that the mind develops through an individual's interactions with the material world around it. Consequently, (inter-) individual differences in e.g., perception, imagination, language processing, or aesthetic judgement, as well as in neuronal activity measured in the sensorimotor cortex are understood as having evolved from these interactions. The activation of interoceptive and motor processing networks during these higher cognitive tasks then works as an indicator for the degree of bodily groundedness of a function.
Studies of developmental changes of embodiment phenomena based on the agency approach are rare, with exceptions often focusing on action perception and speech development during infancy and early childhood (Wellsby and Pexman, 2014; Gredebäck and Falck-Ytter, 2015; Fuchs, 2016b; Gottwald et al., 2016; Inkster et al., 2016; Gredebäck, 2018; Loucks and Sommerville, 2018). However, a number of studies examines changes of embodiment phenomena on a much shorter timescale due to e.g., training effects, therapeutic interventions, or short-term manipulation and impairment of motor capabilities (e.g., Koch et al., 2008; Marasco et al., 2011; Meugnot et al., 2014; Kuehn et al., 2018). It is likely that the mechanisms underlying short-term plastic changes of embodiment processes overlap with those underlying the longer-term developmental changes (Wenger et al., 2017). To fully bridge these timescales, however, agency approaches need to be integrated with environmental approaches.
Environmental approaches to embodiment, on the other hand, explicitly examine the developmental outcome related to the environmental impact under study. The goal is to trace the underlying biological processes, which lead to the observed associations in longitudinal epidemiological data (Hanson and Gluckman, 2008; Rutter, 2016; see also references in Table 1). This “archeology” (Hertzman, 2012, p. 17163) digs into different biological layers to ultimately identify differences at the level of DNA methylation or protein activity and gene expression. Although environmental approaches to embodiment assume that the incorporation or embedding of experience potentially takes place at multiple time-points, they often register only single events (preferably in early childhood) and their impact on a single biological level (e.g., the genetic and epigenetic level: Godfrey et al., 2007; Caspi et al., 2010). Only rarely, dynamic cross-level transformations of incorporated experiences are studied. This is mainly due to method- and data-related constraints. It implies, however, that we register only main tendencies and might miss most of the environmental impact and biologically embedding of dynamic experiences over the lifespan. In most cases, the “digging process” is limited to the final time-point and one biological condensate of interest.
In this way, environmental approaches often imply a direct causal link between an environmental event and a behavioral pattern, differences in psychological functions, or a mental health outcome. However, there is limited evidence for cases in which an input early in life directly, exclusively, and irreversibly affects the long-term outcome. More often we must assume that the biological foundation undergoes several dynamic developmental processes throughout an individual's life. Also, the mechanisms underlying acute activation of embodied experiences might differ from those grounding the long-term developmental pathways which channel the activation outcome. An experience may be embodied in a way that shapes a developmental pathway, which is then channeling but not determining the acute activity patterns, such as determining hormonal setpoints in the stress response system. This is critical, as any identified mediators and intermediate developmental stages of embodiment processes open up targets for interventions.
Table 2 summarizes developmental theories and their critical propositions for developmental embodiment research.
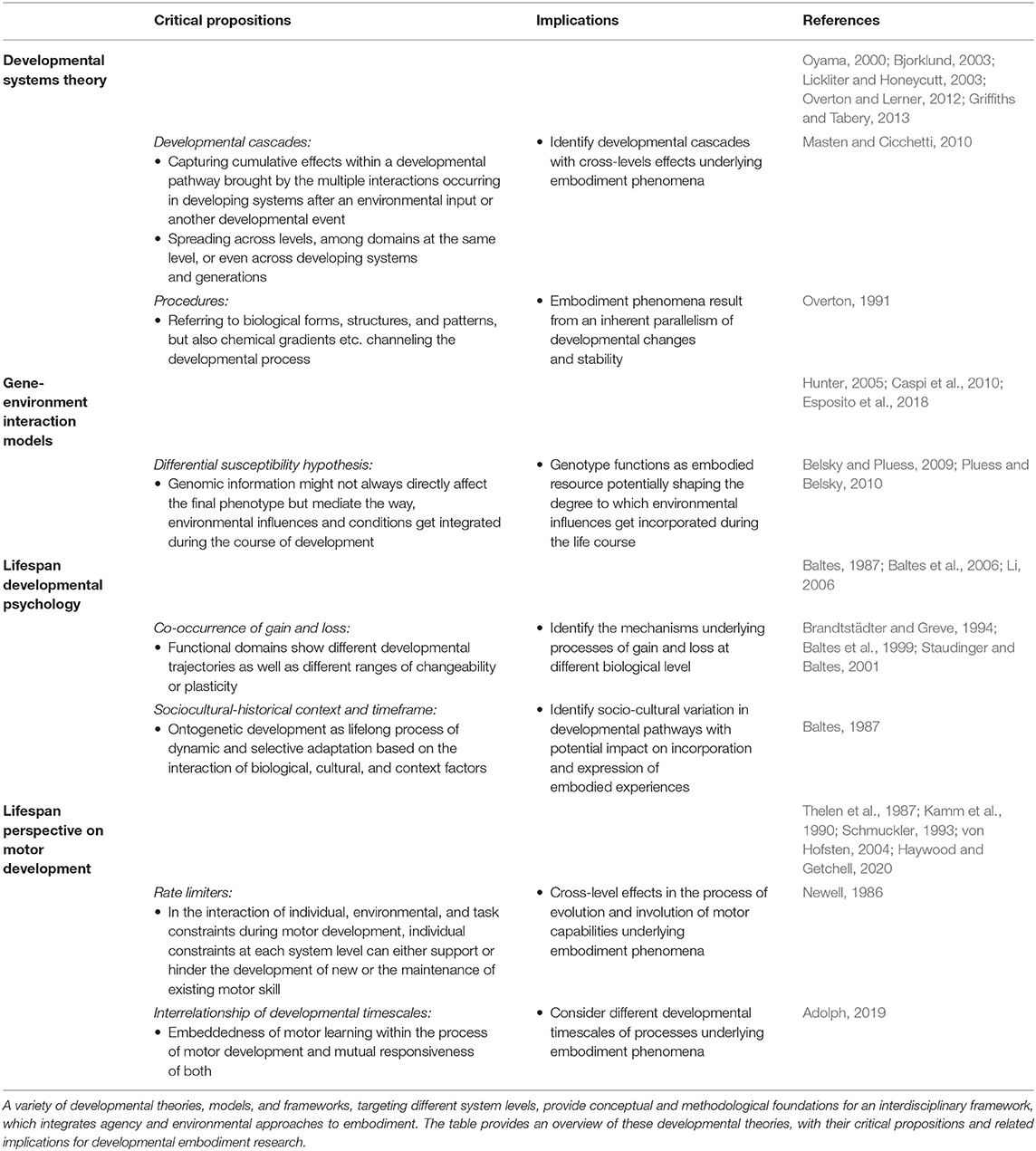
Table 2. Lifespan developmental theories and their critical propositions and implications for developmental embodiment research.
Developmental Embodiment Research: Cross-Disciplinary Examples
Practical examples of interdisciplinary embodiment research are still rare. Among these examples, only a few explicitly address embodiment processes from a developmental perspective. Here, we present a small selection of them, which shows the range of disciplines and research fields for which a developmental perspective of embodiment provides a useful platform. We selected these examples to illustrate the productivity but also some of the challenges of our proposed framework. Because of the many-faceted conceptualization of embodiment in the life sciences, the following examples can only be spotlights, highlighting different levels and areas of embodiment research, where, e.g., a developmental perspective is already employed, but needs refinement and more systematic standards across timepoints and experimental systems (see section Example 5: Epigenetic Mechanisms as Biomarkers for the Impact of Early Life Stress on Mental Health), or where such a perspective would help integrate data (see sections Example 1: Age-Related Cognitive Decline Impacts Motor Control and Example 4: The Developmental Impact of Limited Interoceptive Perception in Autism Spectrum Disorders) or provide additional criteria for competing theoretical explanations (see section Example 3: The Role of Sensorimotor Systems in Abstract Concept Representation and Example 7: Modeling Motor and Cognitive Development With Robots). Also, the examples described in the following demonstrate that the integration of timescales and levels needs to be case specific for each embodiment phenomenon, since, depending on the methods and data available, studying cross-level effects and indicators of long-term changes are quite different across disciplines and research topics.
Example 1: Age-Related Cognitive Decline Impacts Motor Control
One first example, for how current interdisciplinary embodiment research profits from an explicit developmental perspective, is motor decision making. Decision making research has a long tradition in psychology. However, its relevance for understanding changes in movement coordination of everyday activities such as reaching and grasping has only recently been acknowledged: Cisek (2007), Cisek and Kalaska (2010), and Cisek and Pastor-Bernier (2014) highlighted the embodied nature of motor decision making, and its temporal dynamics during movement planning and control in a series of theoretical papers. Following this approach, Gallivan and Chapman (2014), Gallivan et al. (2018), Krüger and Hermsdörfer (2019), and Salzer and Friedman (2019) provided empirical evidence for these assumptions by showing changes in the execution of reaching movements under different conditions for motor decision making. It has been suggested that the perceived or expected biomechanical costs of a movement can reverse decisions to reach to particular targets (Burk et al., 2014) and can bias perceptual decision making when coupled to motor responses (Hagura et al., 2017). Thus, embodiment research, spanning the levels of neural, sensory, and motor activity, as well as complex psychological function and behavior, has advanced the cross-disciplinary understanding of decision making processes. Still, what is largely missing at present is the integration of empirical evidence on lifespan developmental changes of cognitive, perceptual and motor processes, stemming from the different research disciplines, into (motor) decision making theories: i.e., how age-related changes in cognitive and perceptual decision making, due to age-related changes in cognitive functioning and underlying neural networks (Mata et al., 2007; Eppinger et al., 2011; Kurnianingsih et al., 2015), relate to age-related changes in movement coordination and motor function (Verrel et al., 2012; Krüger et al., 2013), and vice versa. A developmental perspective on embodiment would allow for this integration by highlighting the dynamic and mutual interrelationship between motor and cognitive functioning across the lifespan, potentially also providing hints for the origins of the increasing inter-individual variability in cognitive and motor functioning with increasing age. In addition, it opens new avenues for research in the context of neurorehabilitation, since it underlines the need for multi-professional interventions to alleviate motor and cognitive impairments after e.g., stroke.
Example 2: Motor Expertise Changes Perception and Cognition
In a different context, the interaction between motor skills, perception and cognition, and their neural basis is already studied from a developmental perspective, but on a much shorter timescale than lifespan development: In research on motor expertise, a developmental perspective has been adopted to measure how the adult brain changes during motor skill learning and physical training (Wenger et al., 2017). This approach of observing the dynamics and patterns of neuroplasticity during motor learning might contribute to explaining embodiment phenomena found in this context. Several studies convincingly showed that motor expertise changes perception and cognition. Movements which had been extensively trained were more readily recognized in subsequent visual discrimination tasks (Casile and Giese, 2006; Aglioti et al., 2008). Importantly, these perceptual improvements cannot be explained by visual experience alone, but suggest motor learning-induced plasticity in the sensorimotor system to affect perception and cognition as well. This is in line with several theoretical assumptions. One of them is the common coding account which considers overlapping representations of action and perception (Prinz, 1997). It also fits with the notion that neural networks for motor control have evolved to contribute to both motor actions and cognition (Ptak et al., 2017), which, again, is in line with a dynamic systems approach to cognitive development (Thelen, 2000) and with the predictive coding framework (Kilner et al., 2007). From this perspective, it is a pressing research question whether and how neuroplasticity in the sensorimotor system accounts for the development of special cognitive and perceptual skills in movement experts.
Example 3: The Role of Sensorimotor Systems in Abstract Concept Representation
A developmental approach could also provide valuable new insights into the mechanisms underlying the representation of abstract concepts. In particular, by studying children's acquisition of abstract vocabulary we can test claims about the role of sensorimotor systems in knowledge representation (for a review see Pexman, 2019). Currently, at one end of the spectrum, amodal theories posit that knowledge is represented symbolically, which means that concepts are distinct from the ways we experience them (e.g., Quillian, 1969; Pylyshyn, 1985). At the other end of the spectrum, strongly embodied theories posit that knowledge is grounded in sensory, motor, and emotion systems (e.g., Glenberg and Gallese, 2012; Glenberg, 2015). Between these poles lie multimodal or hybrid theories, which posit that knowledge is represented in many ways (e.g., language, emotion, introspective, and sensorimotor) and that different kinds of information are important for different types of concepts (e.g., Barsalou et al., 2008; Borghi et al., 2019). These theories have been tested extensively in the context of research on adult concepts and language processing, with much recent support for multimodal theories (for a review see Zwaan, 2014). The underlying predictions about the acquisition of word meanings during language development in children have only begun to be tested (Wellsby and Pexman, 2014), with a handful of recent studies testing the validity and area of application of two competing theoretical proposals, the emotion bootstrapping proposal (Ponari et al., 2017, 2020; Lund et al., 2019) and the language competence proposal, suggesting that future studies should more carefully consider children's acquisition of different types of abstract words (Lund et al., 2019). These recent studies provided some initial insights, but their cross-sectional designs and methods offer limited inferences about children's representations of abstract concepts. Studies have not yet systematically explored the predictors and outcomes of abstract vocabulary acquisition.
Example 4: The Developmental Impact of Limited Interoceptive Perception in Autism Spectrum Disorders
Selfhood and emotions have long been understood to be grounded in representations of the physiological state of the body (James, 1994; Damasio, 1999; Critchley et al., 2004; Craig, 2009b; Seth, 2013). More recently, research into interoception has demonstrated the extensive significance of our inner bodily signaling systems for decision making, time perception, emotion processing, and behavior in general (for review, see, e.g., Herbert and Pollatos, 2012; Seth, 2013). Consequently, for disorders that involve disturbances of self-representations (e.g., psychosis), emotional processing (e.g., alexithymia, anxiety and mood disorders), or with strong somatic components (e.g., depression and eating disorders), a primary dysfunction in the perception and regulation of body states has been considered (Paulus and Stein, 2010; Herbert and Pollatos, 2012; Stephan et al., 2016; Petzschner et al., 2017; Khalsa et al., 2018). An example are autism spectrum disorders (ASD), a spectrum of neurodevelopmental conditions characterized by lifelong difficulties in social and emotional functioning (among other impairments, Frith, 2014). ASD have been hypothesized to be related to interoceptive failure (Quattrocki and Friston, 2014). However, studies investigating interoceptive abilities in adults with ASD have yielded mixed results (Garfinkel et al., 2016; Shah et al., 2016; Gaigg et al., 2018; Palser et al., 2018). Critically, these discrepancies can potentially be resolved by adopting a developmental perspective: Quattrocki and Friston (2014) suggest that during a critical period of early childhood development, interoceptive signals need to be contextualized to support a typical development of emotional awareness and social attention. In particular, the association of interoceptive signals of warmth and satiety with an infant's caregiver serves as the basis for attachment behavior and endogenous social attention. The authors theorize that a difficulty in interpreting one's own bodily signals early on, potentially caused by abnormal regulation of the oxytocin system in ASD, prevents such associative learning between interoceptive and exteroceptive (social) cues, and leads to impairments of emotional awareness and social interaction later in life. Consistent with these ideas, recent studies support a diminished interoceptive accuracy in children with ASD (Nicholson et al., 2019), and specific impairments in the integration of interoceptive and exteroceptive information (Noel et al., 2018). However, data on interoceptive abilities in infants are scarce (although suitable experiments have been proposed) and very little is known about how these abilities develop across the lifespan (Murphy et al., 2017).
Example 5: Epigenetic Mechanisms as Biomarkers for the Impact of Early Life Stress on Mental Health
One example for the potential but also the difficulties to identify links between different levels of embodiment when following an environmental approach is research investigating epigenetic mechanisms underlying long-term mental health effects of early life stress. Data from several longitudinal studies, clinical samples, as well as animal research support the link between early life stress and mental health (see the review by Provençal and Binder, 2014). Although some assume that the effects of early life stress only impact the individual after multiple stressful experiences (Binder et al., 2008; Danese et al., 2011; Zannas et al., 2015), there is consensus among researchers that early life stress gets somehow biologically embedded or embodied during critical periods in a way that mediates later effects. A growing body of research has identified stressor specific effects and effects of different stressor intensities (Bock et al., 2015; Lux, 2018; Aristizabal et al., 2019), as well as several factors of resilience or reversibility (Harris et al., 2016; Serpeloni et al., 2019; Hartmann and Schmidt, 2020, Francis et al., 2002). The main challenge for the research field is to determine the causal pathway that integrates effects of different embodiment levels (from molecular to behavioral and cognitive) at several critical time points across the lifespan, and especially in early life periods.
Here, one line of research focuses on epigenetic modifications as relatively stable intermediate level, coordinating genetic constitution, and environmental signals, as indicated by animal studies (Weaver et al., 2004; Murgatroyd et al., 2009; Franklin et al., 2010). Although epigenetic modifications following early life stress are reported repeatedly (for a review see Aristizabal et al., 2019; with focus on human studies Vaiserman, 2015), mechanistic links between these and other levels of embodiment including the stress hormone system and stress and emotion regulating neural networks are still not identified (Aristizabal et al., 2019). Instead, contradictory findings complicate the picture. To make sense of the current findings, the field works at developing integrative models spanning different time-points of embodiment and highlighting critical periods, during which exposure to adverse environments and stress impacts developmental pathways much stronger than during other periods over the lifespan (Lupien et al., 2009; Bock et al., 2015; Non et al., 2016; Non, 2021). In addition, more research is needed to distinguish between long-term epigenetic modifications observed following early life stress and those related to acute stress exposure later in life, to determine the importance of developmental timing and cumulative effects. Trans- and inter-generational effects in mammals and humans are implicated but very challenging methodologically to determine, and thus still under controversial debate (Horsthemke, 2018; Lacal and Ventura, 2018; Perez and Lehner, 2019).
Example 6: Identifying Sensitive Periods for the Incorporation of Embodied Experiences
An example of research that could address the question of critical periods using a developmental framework are experiments that can establish causation and go beyond traditional observational studies. For example, Provençal et al. (2019) tested epigenomic effects of exposing fetal-derived neurons in vitro to stress hormones (glucocorticoids) across different time periods of exposure, and even how they prime future gene expression responses to stress. While experiments like these are divorced from interactive effects in the body, they can be a first step toward establishing if and when critical periods for stress exposure may alter the epigenome in the relevant tissue of interest. These experiments, of course, test only short-term early life embodiment and would benefit further by integrating findings with longitudinal human studies to see if the same epigenetic effects last throughout the life-course across accessible tissues and contribute to long-term mental health effects. For this, the research field would clearly profit from knowledge about the developmental dynamics of embodiment processes. Although epigenetic mechanisms are still the primary focus, more complex cross-level effects of stabilization and mediation, especially between epigenetic modifications, the stress hormone system, and the formation of neural networks have been identified as promising targets for this line of research (Lux, 2018; Aristizabal et al., 2019; Fogelman and Canli, 2019, Hartmann and Schmidt, 2020).
Example 7: Modeling Motor and Cognitive Development With Robots
In the field of human robotics, the turn to embodied cognition theories initially went against the information processing paradigm of artificial intelligence (AI) (see Hoffmann and Pfeifer, 2018). Until then, AI units constructed according to the information processing paradigm showed tremendous success in pattern recognition and human-like learning behavior, but they were strongly limited by the available processing capacity. The turn to embodied cognition theories within robotics was supported by the production of simple robots imitating the anatomy of living organisms and showing stable and sophisticated motor and sensory behavior without the need of complex information processing systems. One of the most striking examples is the passive dynamic walker, inspired by the anatomy of human legs, which is able to walk stably and smoothly on a plain surface due to its mechanical properties and without complex processing of movement control (McGeer, 1990; Collins et al., 2005). In a similar way, Brooks developed robots with simple parallel sensory processing units, partially hierarchically clustered, which navigate successfully within their environment without the need of complex representation (Brooks, 1991). However, these example robots, as impressive as they are, are restricted to on-time processing of the ‘here and now' and not able to learn from previous experiences. A developmental perspective on embodiment, combining the embodied cognition approach with machine learning and similar algorithm-based self-controlled processing shows promising potential for overcoming some of the boundaries inherent to each approach (Bongard et al., 2006; Sloman, 2009; Hoffman, 2012; Hoffmann and Pfeifer, 2018), with first computer models (Hoffman, 2012) and prototypes being developed. These robots use mechanically inbuilt embodied information to guide motor control and sensory input to reduce processing capacities necessary for interactions with the environment, which are then free for complex, i.e., capacity demanding cognitive processes, e.g., learning.
In addition, human robotics research based on a developmental embodiment perspective also provides a platform and model for the study of human cognition and motor development by allowing to control and observe the functional elements as well as the developmental processes to a degree which is not possible in living organisms (Pfeifer and Bongard, 2006; Hoffmann and Pfeifer, 2018). For example, based on a computational approach to developmental systems neuroscience, Schöner et al. (2018) simulated a simple neural dynamic model of movement generation, serving as platform for discussing infants' developmental challenges as they learn to reach for objects. Also, based on this model, they were able to construct a neural inspired robot imitating the neural processes underlying the reaching behavior (Tekülve et al., 2019). Although such modeling approaches are limited to engineering and computing capacities, they provide a potent tool to test hypotheses between different levels of embodiment, especially between the neural network level and the level of sensorimotor processing and motor actions.
Toward an Integrative Framework for Developmental Embodiment Research
As the previous examples show, developmental embodiment research focuses on cross-level effects underlying developmental processes, and a lifespan perspective to overcome restrictions of previously used approaches within their disciplines. An interdisciplinary framework acknowledging this research approach has the power to provide a matrix and platform for specific empirical studies or experiments on embodiment processes and phenomena, executed based on discipline specific standards and methods. Such a framework, as we propose in the following, maps out connections between levels of embodiment, with the goal to identify and address white spots across the map which, when filled, further complete the picture of a specific embodiment phenomenon. In this section, we will first introduce the main pillars of our framework and then outline important steps to bridge different timescales and levels of embodiment in cross-disciplinary embodiment research.
Environmental and agency approaches represent complementary perspectives. As Figure 1 illustrates, environmental and agency approaches to embodiment represent complementary perspectives in this endeavor: While environmental approaches focus on the process of incorporation of experiences over the lifespan (green arrow), agency approaches focus on the process of expression of embodied capacity within a specific behavior, emotional state or functional ability, simultaneously expressing and, through the action etc., shaping bodily preconditions (orange and yellow arrow). Both complementary, but analytically distinct approaches are interconnected by developmental processes along the individual's developmental timeline (gray-colored circular arrow).
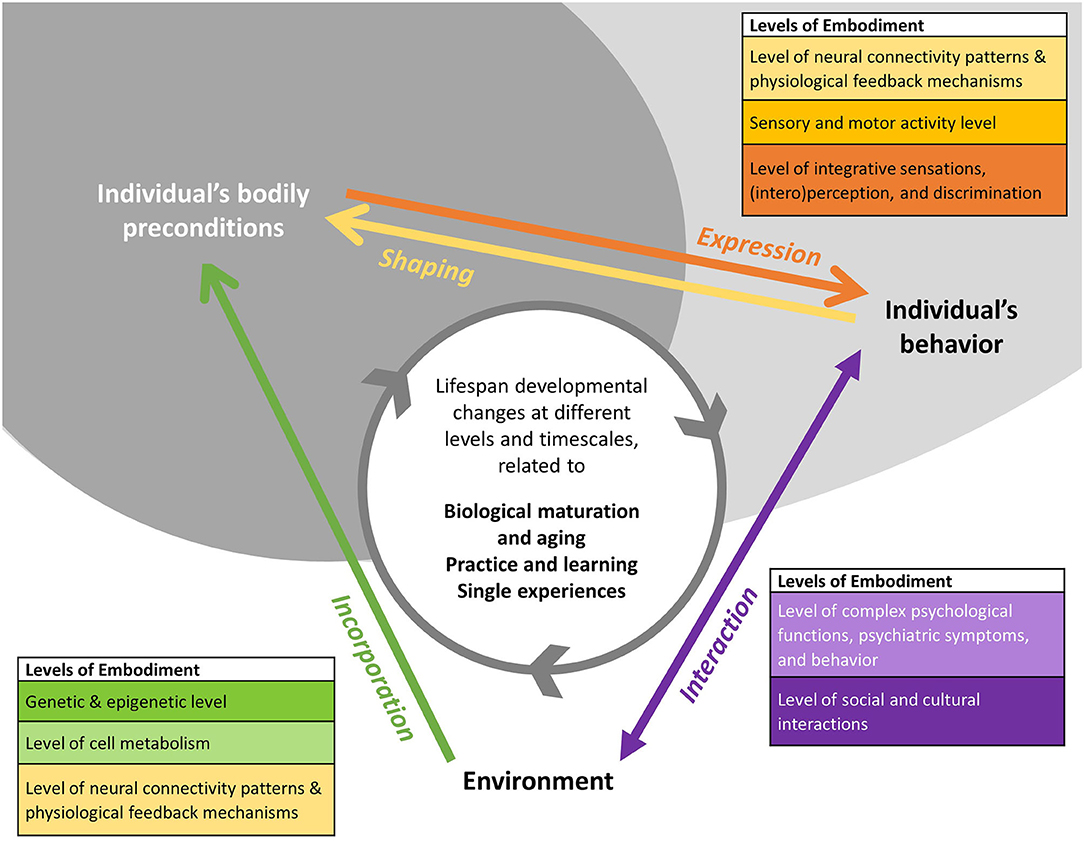
Figure 1. Developmental perspective on embodiment. The figure illustrates how different conceptualizations of embodiment relate to each other from a developmental perspective. Arrows represent the different embodiment processes with the colors indicating the physiological and functional levels at which they are analyzed. The direction of the arrows indicates the perspective on the person-environment relationship. While environmental approaches focus on the process of incorporation of experiences over the lifespan (green arrow), agency approaches focus on the process of mobilization of embodied experiences and knowledge (purple arrow) within a specific behavior, emotional state or functional ability, simultaneously expressing and, through the action etc., shaping bodily preconditions (orange and yellow arrow). The developmental perspective emphasizes that both types of processes change the person-environment relationship. Lifespan changes of bodily preconditions, occurring at different timescales, result in lifespan developmental changes of embodiment phenomena and processes (gray colored circular arrow).
Multi-level approach. In addition, Figure 1 illustrates the different physiological and functional levels potentially involved in the processes of incorporation, shaping and expression of embodied experiences. At the moment, these levels are often studied separately in different disciplines and by different theoretical schools, depending on their respective concept of embodiment. An explicit developmental perspective, relying on critical propositions of developmental theories, shifts the focus on the developmental processes interconnecting the mechanisms underlying a specific embodiment phenomenon at the different levels.
For example, from a developmental embodiment perspective, epigenetic modifications as well as the underlying genotype could be understood as developmental resources. These resources shape the way and degree to which environmental influences are incorporated during the life course and impact developmental outcomes, cumulating, for example, in disease vulnerability or resilience. These then represent some of the underlying bodily preconditions, for example, in a situation of acute mental distress, potentially shaping the symptomatic re-/action to a stressful situation. Pluess and Belsky (2010) discussed these observations as differential susceptibility to environmental signals (see Table 2) and environmental sensitivity (Pluess, 2015), Weaver et al. (2004) framed it as epigenetic programming. The impact of molecular changes at the epigenetic level on the adult phenotype, including disease symptoms and responsiveness to therapy, is a pressing research question. Specifically, at the genetic and epigenetic level (greenish level in Figure 1), environmental epigenetics (Weaver et al., 2004; Zhang and Meaney, 2009; Bollati and Baccarelli, 2010) and studies of gene-environment interactions (Caspi et al., 2003, 2010; Risch et al., 2009; Culverhouse et al., 2018) analyze molecular long-term (ontogenetic) effects of embodied experiences. In addition to one-time severe impacts, such as traumatic events or toxin exposure, also multidimensional and enduring environmental signals are studied, such as enriched environments (Zhang et al., 2018), bullying (Mulder et al., 2020), racial discrimination (Brody et al., 2016), socio-economic status (Needham et al., 2015; McDade et al., 2019), social deprivation (Non et al., 2016), and gender experiences (Cortes et al., 2019). For these fields, our framework would provide the currently missing cross-disciplinary matrix to integrate the different time-points and levels under study.
Accounting for change and stability. Further, from a developmental embodiment perspective, we conceptualize embodiment phenomena as resulting from a balance of developmental change and stability, which depend on the presence, strength, and timing of the environmental signal. Coming from Developmental Systems Theory, which emphasizes that the developmental system consists of dynamic feedback loops, Overton (1991) introduced the term procedures to characterize temporarily stabilized parts of the developmental systems present in embodiment phenomena (see also Table 2). Bones, grown structures and patterns of tissue, muscle constitutions and setpoints within metabolic or hormonal feedback loops, neural pathways and networks, epigenetic modifications but also automized behavioral patterns potentially function as established and stabilized procedures sculpting developmental pathways. Although procedures continuously need to be maintained within the developing system, we can classify them as embodied when the energetic costs to transform a procedure outweigh those to stabilize it, keeping in mind that the balance between energetic costs for stabilizing a procedure and transforming it might change during the course of the lifespan.
For example, the hormonal feedback loops underlying the stress response are established early in development. During this critical developmental period, set points of up- and downregulation of the stress response in presence of an acute stressor are established depending on the individual metabolic conditions and stress experiences during this period. These are then stabilized, however, the stabilization might be challenged by following life experiences, such as traumatic events, hormonal imbalances during adolescence, pregnancy, chronic stress, or aging, which all might lead to changes of the original set-point. The exact mechanisms of stabilization and change of these set-points are still only partially known, yet, it is assumed that they contribute to stress-related mental health impacts.
Another example would be establishment of neural pathways underlying automatized word recognition in reading. The ability to learn how to read depends on age-dependent neural and cognitive development with only some degree of inter-individual variability indicating dependence on other bodily embedded developmental processes. Once the basic ability to learn how to read is developed, instructions and practice are needed until the stage of automized word recognition is reached. The neural mechanisms, such as synaptic sensitization and neural pathway stabilization, underlying this automatization process are likely similar to (and may even rely on) processes of automatization of other motor and sensory activities, yet, again, they are still partially known. Their further elucidation would contribute to our knowledge on the mechanisms underlying various variants of dyslexia as well as possible impacts of strokes and subsequent rehabilitation therapy.
Developmental embodiment research would address these mechanisms with a multi-level cross-disciplinary approach and lifespan perspective. It would also address that the pathways of embodiment are then potentially two-fold, as can be traced in Figure 1, via a more passive incorporation (green arrow) or via the expression-shaping cycle (yellow and orange arrows) mediated by the individual's interaction with the environment (purple arrow). As both are depending on each other, with acute activation necessary for change and maintenance on the one side and incorporation setting some of the preconditions for the activation process on the other side, overcoming the current practical divide in embodiment research between environmental approaches and agency approaches is crucial to further advance our understanding of these processes. Interconnecting them via a developmental perspective would allow to assess criteria and thresholds for change and stability at a specific level (of, for example, genetic and epigenetic activity, cell metabolism incl. hormonal activity, neural network activity, sensory, and motor activity) within different developmental periods across the lifespan.
Cross-level effects cumulating in developmental cascades. An explicit developmental perspective on embodiment, building upon critical propositions of developmental theories, also highlights the cross-level dependencies and effects of embodiment processes and phenomena. According to the life span perspective on motor development (see, for example, Haywood and Getchell, 2020) skilled motor behavior follows from a self-organization process of multiple system levels within an individuum, e.g., muscular, skeletal, neural, cognitive, etc., referred to as “individual constraints” (Newell, 1986). Based on this assumption, it follows that, for developmental motor behavioral changes to occur, all individual constraints have to be developed to a required level. Here, the proposition that individual constraints can act as rate limiters, i.e., either restricting or facilitating the development or decline of motor skills, implies cross-level dependencies of development (see also Table 2). Masten and Cicchetti (2010) discuss such cross-level effects under the term developmental cascades (see Table 2). Developmental cascades capture cumulative effects within a developmental pathway brought by the multiple interactions occurring in developing systems after an environmental input or another developmental event. The key characteristic of such a cascade is that the effect spreads across levels, among domains at the same level, or even across developing systems and generations (Masten and Cicchetti, 2010, p. 492). From the perspective of developmental embodiment research, it would then be the goal to identify developmental cascades with cross-levels effects underlying embodiment phenomena. This also stipulates identification of levels involved and affected within a specific embodiment phenomenon, identification of transmission hubs between these levels as well as identification of time-points of transmission. These differ for the particular phenomenon under study, e.g., for the biological embedding of early adversity and its long-term impact on mental health, the way motor experiences ground the development of cognition and emotion processing, and how imagination techniques improve motor rehabilitation outcomes after a stroke.
Identification of transmission hubs. Trying to identify developmental cascades when taking the systemic and dynamic character of biological processes seriously, as from a developmental systems perspective, would require to account for all levels of the system at every time-point during development. However, such a comprehensive approach is not feasible in concrete empirical studies, even with cross-disciplinary collaborations addressing different levels, timescales, and data modalities. Therefore, we propose to focus on the identification of transmission hubs between levels of embodiment. Transmission hubs constitute the molecular, physiological, cognitive, etc. structures which participate in the transmission of signals related to a particular embodied experience across levels, such as, e.g., neural networks underlying motor execution that are also involved in imagination and cognitive processing, neural and hormonal correlates of interoception in emotion processing and related disorders (see section Developmental Embodiment Research: Cross-Disciplinary Examples, Example 4), molecular feedback loops underlying the regulation of synaptic plasticity, neurotransmitter activity, and the stress response, as well as processing of biomedical knowledge influencing self-perception, symptomatic experiences, and mental health. These transmission hubs are characterized by their critical role to enable or block transmission of developmental changes between levels, thereby stipulating and canalizing embodiment processes. Importantly, with respect to the developmental perspective, transmission hubs are not fixed across the lifespan, but change due to developmental processes, e.g., in the degree of plasticity, the involved biological, and functional levels of transmission, the signal intensity needed to induce transmission. They are also likely involved in the constitution of critical and sensitive periods but not limited to them.
Accounting for gain and loss. From research in the perspective of lifespan developmental psychology, we draw on the notion that gain and loss always occur together in ontogenetic development (Baltes, 1987) for our integrative framework for developmental embodiment research (see also Table 2). Thus, in addition to analyzing growth and maintenance as well as recovery and resilience, studying the regulation of loss is equally important. While lifespan developmental psychology focuses on the developmental consequences of this selective channeling process, developmental embodiment research additionally aims at identifying the mechanisms underlying these processes at different biological levels.
Functional domains differ in their developmental trajectories. Also in reference to research in the perspective of lifespan developmental psychology, we account for the notion that different functional domains have different developmental trajectories across the lifespan, which also differ in their range of changeability or plasticity and even between individuals with comparable functional outcome (Brandtstädter and Greve, 1994; Baltes et al., 1999; Staudinger and Baltes, 2001). As a methodological consequence, person-centered (holistic) and function-centered approaches need to be combined to study these developmental trajectories (Baltes et al., 1977; Baltes, 1987). Although a function-centered approach will probably be used most in developmental embodiment research, a person-centered approach might become more relevant when the field expands into translational research and when focusing on inter-individual differences in the expression of embodiment phenomena.
Accounting for sociocultural-historical changes. Finally, a developmental embodiment perspective reinforces the necessity to account for sociocultural-historical changes and their impact on the bodily preconditions of psychological functions. This includes the effects of socio-cultural contexts (family, school, work, etc.), historical changes of educational and professional systems, cultural norms, (religious and other) traditions, as well as historic events, such as war periods and collective traumatic events. Some examples following an environmental approach to embodiment are efforts to identify epigenetic mechanisms underlying the long-term impact of traumatic events such as the attacks of 9/11 (Kuan et al., 2017) or war crimes (Ramo-Fernández et al., 2015). Another example in this perspective are studies on changing neural networks and cognitive performance in children after entering school (Brod et al., 2017). Examples following an agency approach to embodiment are analyses of bio-looping effects of cultural knowledge and resources including scientific concepts and how they influence the active construction of self-perception patterns and identity, emotion regulation, and health and body related behavior (Seligman et al., 2015; Kirmayer and Gómez-Carrillo, 2019).
Thus, for developmental embodiment research, considering lifespan dynamics of embodiment does not only entail the consideration of different timescales of development, but also the consideration of different processes, which contribute to these developmental changes and the different timescales at which these processes act. Reconstructing the developmental cascade therefore requires integrating data generated with different experimental settings and study designs, at different time-points, at different levels, and across the involved disciplines.
Bridging Timescales of Embodiment
With our framework for developmental embodiment research, we explicitly aim at bridging the different timescales of embodiment focused on either by agency or environmental approaches (see Figure 1).
First, we emphasize studying developmental processes related to embodiment phenomena along different timescales, foremost across the whole lifespan. Due to the underexplored lifespan perspective, buffering effects and functional changes during development are often underestimated, as shown, for example in research on the biological correlates of resilience (Feder et al., 2009) and longitudinal studies of stress buffering effects of social support and mental function in old age (Toyama and Fuller, 2020). To identify these, the study of functional gains should be complemented with the study of mechanisms of maintenance and of the regulation of loss, as well as with the search for alternative developmental pathways in studies using inter-individual comparisons. However, shorter timeframes of developmental dynamics also have to be considered as periods in which such alternative developmental pathways are initiated. When considering development as a result of interacting constraints at different system levels within the individual, and between the individual and the environment, single events, as, for example, traumatic experiences or learning processes may lead to immediate changes of developmental pathways, with lifelong consequences for related embodiment phenomena. Thus, depending on the phenomena under study, also shorter timeframes of analysis, e.g., for specific learning processes, or a repeated analysis of shorter timeframes spread out across the whole lifespan, as often used in longitudinal studies, should be considered for being able to identify buffering effects und functional changes across the lifespan.
Second, despite a focus on the whole lifespan, we also need to continue identifying sensitive periods during which experiences are more likely to be embedded. For this, we need to consider that sensitive periods differ across different neural and other physiological systems contributing to motor, cognitive, and brain development. Moreover, the study of sensitive periods, such as the perinatal phase, early childhood, and adolescence, needs to be complemented by the study of subsequent stabilization periods, and phases of recession and degeneration in later life. Only when the embodied experience, as acquired through interaction with the environment, is preserved, can it be relied on at a later time-point and can thus have a developmental impact. These stabilization processes might provide promising targets for intervention, especially with regard to later phases of accelerated decline in functioning at multiple behavioral and physiological levels.
Third, for every behavioral outcome, symptom, or function studied in terms of embodiment, commonalities but also differences between the biological processes contributing to the incorporation of experiences and those used for expressing the embodied experiences during action need to be taken into account. This is of particular practical relevance for interventions, which may differ significantly in their structure and outcome depending on whether they target the first or the latter. For example, in the context of mental health, an intervention might aim at increasing resilience (to prevent incorporation of negative experiences), or it might aim at increasing behavioral flexibility and at re-learning after a negative environmental impact (such as cognitive-behavioral interventions), or at interfering neurochemically with the circuit that implements the embodied processes (as pharmacological interventions do).
Fourth, depending on the system level, an evolutionary timeframe needs to be considered. Identification of phylogenetic evolved plasticity and environmental sensitivity parameters for specific target systems and tissue will inform clinical and intervention studies. However, it is important to not confuse the phylogenetic timeframe, which addresses species development at the population level in a co-developing organism-environment context, and the ontogenetic timeframe within the lifespan of an organism. Developmental dynamics of different physiological systems or neural networks will vary between individuals. Identification and description of species-specific developmental periods needs to account for this variability.
Fifth, across these different timescales of phylogenetic developed sensitive periods, their socially and culturally shaped realization during ontogenetic development, and such single short-term experiences with long-lasting effects, we suggest focusing on the transmission hubs underlying developmental processes, which connect processes and functions at different levels with each other and provide transition points within the developmental pathways. One example would be the study of traumatic stress during sensitive periods of brain development contrasted with traumatic stress experienced before or after such a sensitive period. The transmission hubs of interest here would be those hormonal, neural, and molecular feedback loops whose interaction constitute sensitive periods thereby opening up the involved systems for the embodiment of the environmental signal.
Bridging Levels of Embodiment
In addition to accounting for different timescales that underlie the processes of incorporating and expressing embodied experiences, we propose that a developmental perspective allows for connecting different biological and functional levels of embodiment. For that, the involved levels of embodiment have to be clearly differentiated to carve out conceptual and methodological gaps that need to be bridged when studying a specific embodiment phenomenon. Identification of levels is a precondition for cross-disciplinary data integration.
There are multiple ways to differentiate levels of embodiment. For the aim of our proposal—connecting different approaches of embodiment research from a developmental perspective—we differentiate between levels along the methods used to assess, observe, induce, and evaluate changes of embodiment. This is a first step in the process of integrating data from different research fields and approaches with each other, despite the conceptual variability in embodiment definitions.
We propose to differentiate between at least seven levels of embodiment (see Table 3): The genetic and epigenetic activity level (1), the level of cell metabolisms (including proteomics analyses) and single neuronal activity (2), the level of neural connectivity patterns and physiological feedback mechanisms (e.g., hormonal, metabolism related) (3), the sensory and motor activity level (4), the level of integrative sensations, (intero)perception, and discrimination (5), the level of complex psychological functions, psychiatric symptoms, and behavior (6), and the level of social and cultural interactions (7). The number of levels and their distinction are not exclusive. We explicitly encourage understanding them as to be open to adaptations depending on their worth for the respective empirical study and the development of new methods.
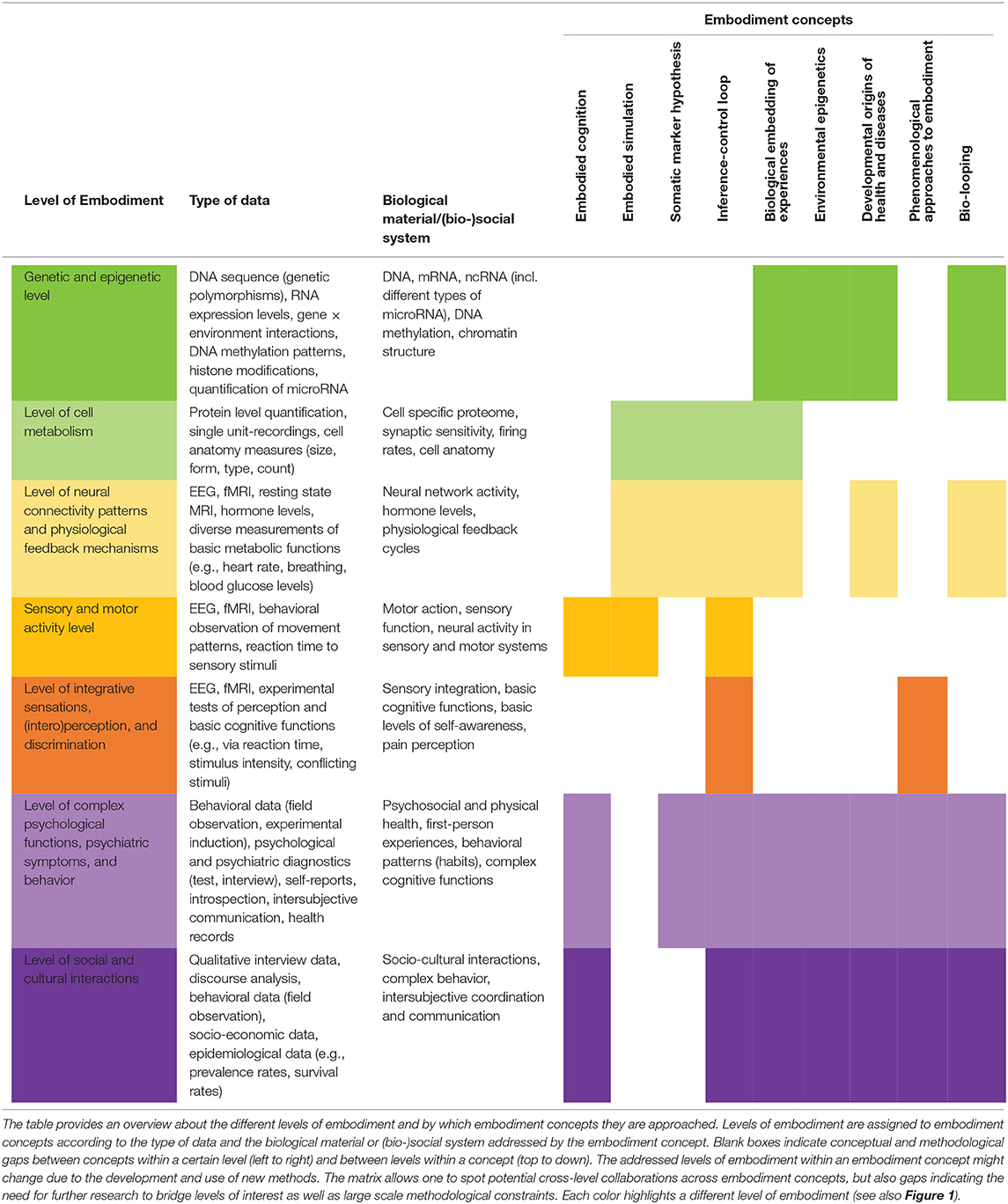
Table 3. Levels of embodiment, types of data, and biological materials or (bio-)social systems, matched with embodiment concepts by which they are addressed.
We further propose to understand these levels as analytical tools rather than empirical entities, with the purpose to identify key processes, which coordinate and translate different biological mechanisms underlying embodiment as well as psycho-social and cultural interactions related to them. The method-based differentiation of these analytical levels also enables to visualize where data acquisition at different levels may be easily combined or exclude each other (for neuroscience/fMRI see Soares et al., 2016; for genomics/proteomics see Tyers and Mann, 2003; Manzoni et al., 2016; Vitrinel et al., 2019). Also, methods which describe embodiment and embodied experiences from different epistemological standpoints that do not map easily onto each other, such as cultural analysis, introspection, and the detection of neural activity or gene expression patterns, can be made explicit in this framework (for an example on embodied memory and social skills see Fuchs, 2016a). This supports the planning process of collaborative research projects spanning across levels.
The variety of methods used to differentiate between levels of embodiment may seem overwhelming to approach from the perspective of single research groups. Moreover, technical restrictions in data acquisition and epistemological gaps between data modalities (e.g., between subjective experience data and neurophysiological data) mean there will always remain blank spaces in the picture. However, we believe that the differentiation between levels provides a clear basis for trans-level collaboration between two or more research groups and even for larger research consortia. In any case, it helps to identify the blank spaces for specific cases of embodiment phenomena. Furthermore, it allows to spot potential transmission hubs between levels of investigation. These transmission hubs are important targets to understand the process of translating incorporation into expression of embodied experiences across the lifespan. Ultimately, they also constitute promising points of intervention.
Bridging different levels of explanations (and data modalities) is a challenge that is inherent to many fields in the life sciences. A popular tool for achieving such bridges is computational modeling. Formal models of processes at different levels and their interactions allow for an in-silico testing of how changes at one level translate into changes at another level. One recent example is the nascent field of computational psychiatry (Huys et al., 2016; Redish and Gordon, 2016), which acknowledges the multi-leveled nature of psychiatric diseases—ranging from the genetic and molecular level, neural circuits, cognition and behavior to the social and even cultural level—and attempts to bridge these levels of analysis using mathematical tools. The even more recent subfield of computational psychosomatics explicitly considers the role of body perception (interoception) and regulation for the understanding and treatment of mental diseases (Petzschner et al., 2017, 2021). Modeling homeostatic setpoints and their interactions with cognition provides a new framework for understanding the interconnectedness of bodily and mental well-being that becomes obvious in the symptom profiles of all psychiatric and psychosomatic diseases. More generally, these approaches hold great potential for studying embodiment phenomena across different levels.
Clarifying Disciplinary Boundaries and Discipline Specific Criteria in Conceptualizing Embodiment and Embodied Experiences
One of the foremost practical challenges for integrative embodiment research, as suggested in the previous section, is that for each scientific discipline, there are different criteria for when an experience counts as embodied according to the different detection methods and data modalities. In addition, these criteria are sometimes ambivalent and need to be the subjects of further conceptual debates. Consequently, these discipline-specific criteria must be clarified for being able to relate different levels of embodiment and, consequently, embodiment approaches to each other.
At the genetic and epigenetic activity level, for example, we could ask whether detection of a functional relevant genetic mutation or its transcription in the target tissue, as indicated by gene expression analysis, represent the same or two different indicators of embodiment. When using epigenetic data, criteria are even more variable, including not only potential quantitative differences of functionally relevant modifications across tissue types but also different epigenetic mechanisms (DNA methylation, histone modifications, RNA interference etc.), which are functionally and hierarchically intertwined. In addition, epigenetic modifications are often evaluated as functionally relevant when they affect gene expression, although the relationship between gene expression patterns and, for example, DNA methylation is still not fully understood (Lea et al., 2018), and there may be a lag in timing before the functional effect is expressed. Thus, criteria for assessing embodiment can be too strict and not accounting for indirect as well as long-term effects of embodied experiences (Aristizabal et al., 2019), even within the research discipline.
For the level of neural activity, neural activation of motor areas either at a single neuron level or at the network level is often used as a criterion of embodiment, for example, in the detection of the fiercely debated “mirror neuron systems.” Here, the activation of motor neurons during the performance as well as the perception of a motor task is used as indicator of an embodied inner simulation or immediate representation of the task (Rizzolatti et al., 1996; Rizzolatti and Craighero, 2004). From a developmental neuroscience perspective, the developmental stage of a neural network, as indicated by age-specific connectivity patterns activated in a behavioral task, also functions as criterion for the type and degree of embodied experiences (Decety and Michalska, 2020). In contrast, for the level of emotion processing and interoception, Damasio et al. (2013), first referred to a single case lesion study only showing the anatomical absence of specialized neural networks as indicator that our emotion processing abilities are grounded in bodily processes. More recently, the interaction of interoceptive and emotional states as well as with perception and cognition is investigated more systematically by testing the impact of visceral signals and their neural processing on emotional, perceptual, and cognitive functions, and vice versa (for overviews, see Critchley and Garfinkel, 2018; Azzalini et al., 2019).
For the level of complex psychological functions, psychiatric symptoms, and behavior, Needham and Libertus (2011), discussing embodiment research in the field of cognitive development, refer to the experience of acting as a main criterion for embodied experiences. Based on studies conducted by Adolph (1997, 2000), which indicate that infants do not transfer their knowledge of surface characteristics, acquired through motor experience in one type of movement (e.g., sitting), to another type of movement (e.g., crawling), Needham and Libertus (2011) conclude that the information about surface characteristics is embodied via motor experiences and not via an abstract cognitive generalization of these characteristics. Furthermore, they illustrate the asynchrony of motor and cognitive development with different results of Piaget's famous cognitive development test of object permanence (the A-not-B error task) based on the behavioral data used to measure the response (gaze vs. pointing/grasping, in 3 to 4 vs. 8 to 10-month-old infants, respectively). Needham and Libertus (2011) argue that, at this early age, the motor experience of pointing and grasping is not yet connected to the visual detection of object permanence indicating different developmental pathways. Most importantly, the different criteria at the behavioral level for different age groups (pointing/grasping vs. gaze) reveal the importance and productivity of a developmental perspective for detecting the role of embodied experiences and the degree of embodiment underlying the cognitive functions under study.
Further, for higher psychological functions such as imagination, associative thinking, and language processing, Körner et al. (2015) introduce three different mechanisms underlying embodiment effects: direct state induction, indicating a direct impact on how humans feel or process information without interference of any other cognitive mechanism, model priming referring to changes in the accessibility of concepts associated with a bodily state, and sensorimotor simulation indicating mechanisms which affect the ease with which congruent relative to incongruent actions are performed. Furthermore, they outline a set of conditions to test which of these mechanisms are involved in an embodiment phenomenon under study (Körner et al., 2015). For example, when the fluency of two competing tasks, which use the same sensorimotor resources, is enhanced by untraining a more fluent action (as in a right- vs. left-handed task), the underlying embodiment mechanism is based on sensorimotor simulation and not modal priming. As elucidating as this is at the level of higher psychological functions, how can we identify tasks, which use the same sensorimotor processes, in the first place (beyond right- vs. left-handed tasks)? This knowledge necessarily precedes the psychological testing conditions. Furthermore, how do we differentiate between these mechanisms when they co-occur in the studied embodiment effect? From a developmental embodiment perspective, a first step could be to trace the occurrence of these different mechanisms during ontogeny as well as to identify the potentially varying underlying neural networks.
Last, at the level of psychiatric symptoms, embodied experiences of e.g., traumatic events, or of depressive or psychotic episodes are indicated by physical symptoms and symptoms of somatization, such as pain, phantom sensations, but also hormonal imbalances and increased inflammation markers (Schnurr et al., 1998; MacLachlan, 2004; Roh et al., 2007; Abbey et al., 2011; Goldsmith et al., 2012; Blumberg and Dooley, 2017; Yuan et al., 2019).
In sum, these examples for different criteria of embodiment at different levels of investigation echo the diversity and variability in concepts of embodiment and conceptualizations of embodied experiences across scientific disciplines. Thus, it is important to clarify the criteria of embodiment for each level and discipline when conducting embodiment research. The examples also show that embodiment is often detected by studying cross-level, and, by such, cross-disciplinary effects (e.g., between motor behavior and neural activity level). Moreover, they demonstrate that the actual task is to relate the effects at each level to each other. This is especially relevant for those innovative cross-disciplinary research areas that study the processes of incorporation and expression of biological embedded experiences across system levels, e.g., in the context of cognitive development, psycho-social well-being, language acquisition and processing, preservation and rehabilitation of cognitive and motor function, and many more.
Conclusion
Embodiment research is at a turning point. The growing amount of data from various studies across a wide range of disciplines and theoretical schools, investigating embodiment phenomena and their role especially in mental processing and functions, highlights the need for an interdisciplinary framework of embodiment research. Innovative research areas such as movement psychology, social and developmental neuroscience, computational psychosomatics, social and behavioral epigenetics, human-centered robotics, and many more, that are facing issues of data integration across different levels of embodiment, would profit tremendously from such a framework. Especially the integration of behavioral data with data from different biological levels, each of which depend on their own developmental timescales and dynamics, is challenging for these fields. In addition, there is a growing need for a cross-disciplinary consensus on level-specific criteria of embodiment. We propose that a developmental perspective on embodiment is able to provide a framework for overcoming such pressing issues, providing analytical tools to link timescales and levels of embodiment on a case-by-case basis, uncovering the underlying developmental processes, and providing a platform to clarify and, ultimately, bridge disciplinary boundaries among the involved research fields.
The proposed framework is not intended to serve as a guideline for one comprehensive embodiment research project, but to serve as a foundation for structuring a highly interdisciplinary research field and to allow for conceptual anchor points for interdisciplinary research endeavors. Building on both environmental and agency approaches to embodiment, as well as key concepts of developmental theory, the framework motivates the question of how a specific expression of embodied experiences relates to the process of incorporation of these experiences, and vice versa, based on the underlying developmental processes. The way to reconstruct these interrelations will be specific for each embodied function, and it will have to take into account not only the rise of a function, but also its maintenance and the regulation of loss. The developmental perspective allows to, first, connect different timescales of embodiment based on function-specific developmental pathways. It, second, allows to relate different system levels involved in embodiment processes to one another as they develop over the lifespan, based on their physiological and functional interconnectedness. Third, it allows to clarify disciplinary boundaries and their related criteria of embodiment, which are set according to detection methods and discipline standards. The translation of different embodiment criteria between levels, e.g., the behavioral level, the level of neural activity, and the genetic and epigenetic level, heavily depends on the knowledge about the specific developmental interconnectedness between these levels and related underlying developmental pathways for each specific embodied function under study. Here in particular, further research is needed, as such translation processes also provide the basis for cross-level data integration.
First cross-disciplinary examples, as presented in section Developmental Embodiment Research: Cross-Disciplinary Examples, already point toward the productivity of such a framework, but expansion to further research disciplines is needed to fill the knowledge gaps hindering an integrative conceptualization of embodiment. For that, we propose researchers should focus on transmission hubs across two or three levels for a specific embodied function or phenomenon, aiming at identifying developmental cascades which enable cross-level effects of embodiment. These studies would depend on the knowledge about the different developmental timescales of embodied functions and sensitive periods for the incorporation of embodied experiences at the different involved levels. Our proposed framework explicitly aims at providing a matrix and platform to bridge these different developmental timescales in the study of specific embodiment phenomena and, thus, has the potential to advance cross-level, cross-disciplinary embodiment research in the short and long run.
Author Contributions
The first draft of the manuscript was written by VL and MK, and all authors contributed to and commented on previous versions of the manuscript. All authors read and approved the final manuscript.
Funding
The Center for Interdisciplinary Research (ZiF), Bielefeld University, funded the workshop Developmental Perspectives on Embodiment, at which the initial proposal of the framework was discussed. This publication was supported by the Open Access Publication Funds of the Ruhr-Universität Bochum.
Conflict of Interest
The authors declare that the research was conducted in the absence of any commercial or financial relationships that could be construed as a potential conflict of interest.
Publisher's Note
All claims expressed in this article are solely those of the authors and do not necessarily represent those of their affiliated organizations, or those of the publisher, the editors and the reviewers. Any product that may be evaluated in this article, or claim that may be made by its manufacturer, is not guaranteed or endorsed by the publisher.
Acknowledgments
We thank Gustaf Gredebäck for his helpful and inspiring comments to earlier versions of this manuscript. This framework was initially discussed at the workshop Developmental Perspectives on Embodiment, which took place in April 2019 at the Center for Interdisciplinary Research (ZiF), Bielefeld University. We thank the participants of the workshop for the insightful discussion and the ZiF for funding and hosting the workshop. We acknowledge support by the Open Access Publication Funds of the Ruhr-Universität Bochum. Finally, we thank Vanessa Vogel for helping with formatting the draft.
References
Abbey, S. E., Wulsin, L., and Levenson, J. L. (2011). “Somatization and somatorform disorders,” in The American Psychiatric Publishing Textbook of Psychosomatic Medicine Psychiatric Care of the Medically Ill, ed J. L. Levenson (Washington, DC: American Psychiatric Pub), 261–289.
Adolph, K. E. (1997). Learning in the development of infant locomotion. Monogr. Soci. Res. Child Dev. 62, I–VI, 1–158. doi: 10.2307/1166199
Adolph, K. E. (2000). Specificity of learning: why infants fall over a veritable cliff. Psychol. Sci. 11, 290–295. doi: 10.1111/1467-9280.00258
Adolph, K. E. (2019). An ecological approach to learning in (not and) development. Hum. Dev. 63, 180–201. doi: 10.1159/000503823
Adolphs, R. (2002). Recognizing emotion from facial expressions: psychological and neurological mechanisms. Behav. Cogn. Neurosci. Rev. 1, 21–62. doi: 10.1177/1534582302001001003
Aglioti, S. M., Cesari, P., Romani, M., and Urgesi, C. (2008). Action anticipation and motor resonance in elite basketball players. Nat. Neurosci. 11, 1109–1116. doi: 10.1038/nn.2182
Aristizabal, M. J., Anreiter, I., Halldorsdottir, T., Odgers, C. L., McDade, T. W., Goldenberg, A., et al. (2019). Biological embedding of experience: a primer on epigenetics. Proc. Natl. Acad. Sci. U.S.A. 117, 23261–23269. doi: 10.1073/pnas.1820838116
Azzalini, D., Rebollo, I., and Tallon-Baudry, C. (2019). Visceral signals shape brain dynamics and cognition. Trends Cogn. Sci. 23, 488–509. doi: 10.1016/j.tics.2019.03.007
Baltes, P. B. (1987). Theoretical propositions of life-span developmental psychology: on the dynamics between growth and decline. Dev. Psychol. 23, 611–626. doi: 10.1037/0012-1649.23.5.611
Baltes, P. B., Reese, H. W., and Nesselroade, J. R. (1977). Life-Span Developmental Psychology: Introduction to Research Methods. A Psychology Press Book. London; New York, NY: Routledge Taylor and Francis Group.
Baltes, P. B., Rösler, F., and Reuter-Lorenz, P. A. (2006). “Prologue: biocultural co-constructivism as a theoretical metascript,” in Lifespan Development and the Brain: The Perspective of Biocultural Co-Constructivism, eds F. Rösler, P. B. Baltes, and P. A. Reuter-Lorenz (Cambridge: Cambridge University Press), 3–39. doi: 10.1017/CBO9780511499722.003
Baltes, P. B., Staudinger, U. M., and Lindenberger, U. (1999). Lifespan psychology: theory and application to intellectual functioning. Annu. Rev. Psychol. 50, 471–507. doi: 10.1146/annurev.psych.50.1.471
Barker, D. J. (1995). Fetal origins of coronary heart disease. BMJ 311, 171–174. doi: 10.1136/bmj.311.6998.171
Barsalou, L.W., Santos, A., Simmons, W.K., and Wilson, C.D. (2008). “Language and simulation in conceptual processing,” in Symbols, Embodiment, and Meaning, eds M. De Vega, A. Glenberg, and A. Graesser (Oxford: Oxford University Press), 245–283. doi: 10.1093/acprof:oso/9780199217274.003.0013
Belsky, J., and Pluess, M. (2009). Beyond diathesis stress: differential susceptibility to environmental influences. Psychol. Bull. 135, 885–908. doi: 10.1037/a0017376
Binder, E. B., Bradley, R. G., Liu, W., Epstein, M. P., Deveau, T. C., Mercer, K. B., et al. (2008). Association of FKBP5 polymorphisms and childhood abuse with risk of posttraumatic stress disorder symptoms in adults. JAMA 299, 1291–1305. doi: 10.1001/jama.299.11.1291
Bjorklund, D. F. (2003). Evolutionary psychology from a developmental systems perspective: comment on Lickliter and Honeycutt (2003). Psychol. Bull. 129, 836–841. doi: 10.1037/0033-2909.129.6.836
Blumberg, M. S., and Dooley, J. C. (2017). Phantom limbs, neuroprosthetics, and the developmental origins of embodiment. Trends Neurosci. 40, 603–612. doi: 10.1016/j.tins.2017.07.003
Bock, J., Wainstock, T., Braun, K., and Segal, M. (2015). Stress in utero: prenatal programming of brain plasticity and cognition. Biol. Psychiatry 78, 315–326. doi: 10.1016/j.biopsych.2015.02.036
Bollati, V., and Baccarelli, A. (2010). Environmental epigenetics. Heredity 105, 105–112. doi: 10.1038/hdy.2010.2
Bongard, J.C., Zykov, V., and Lipson, H. (2006). Resilient machines through continuous self-modeling. Science 314, 1118-1121. doi: 10.1126/science.1133687
Borghi, A. M., Barca, L., Binkofski, F., Castelfranchi, C., Pezzulo, G., and Tummolini, L. (2019). Words as social tools: language, sociality and inner grounding in abstract concepts. Phys. Life Rev. 29, 120–153. doi: 10.1016/j.plrev.2018.12.001
Brandtstädter, J., and Greve, W. (1994). The aging self: stabilizing and protective processes. Dev. Rev. 14, 52–80. doi: 10.1006/drev.1994.1003
Brod, G., Bunge, S. A., and Shing, Y. L. (2017). Does one year of schooling improve children's cognitive control and alter associated brain activation? Psychol. Sci. 28, 967–978. doi: 10.1177/0956797617699838
Brody, G. H., Miller, G. E., Yu, T., Beach, S. R. H., and Chen, E. (2016). Supportive family environments ameliorate the link between racial discrimination and epigenetic aging: a replication across two longitudinal cohorts. Psychol. Sci. 27, 530–541. doi: 10.1177/0956797615626703
Brooks, R.A. (1991). Intelligence without representation. Artif. Intell. J. 47, 139–159. doi: 10.1016/0004-3702(91)90053-M
Burk, D., Ingram, J. N., Franklin, D. W., Shadlen, M. N., and Wolpert, D. M. (2014). Motor effort alters changes of mind in sensorimotor decision making. PLoS ONE 9:e92681. doi: 10.1371/journal.pone.0092681
Bush, N. R., Edgar, R. D., Park, M., MacIsaac, J. L., McEwen, L. M., Adler, N. E., et al. (2018). The biological embedding of early-life socioeconomic status and family adversity in children's genome-wide DNA methylation. Epigenomics 10, 1445–1461. doi: 10.2217/epi-2018-0042
Buxbaum, L. J., and Kalénine, S. (2010). Action knowledge, visuomotor activation, and embodiment in the two action systems. Ann. N. Y. Acad. Sci. 1191, 201–218. doi: 10.1111/j.1749-6632.2010.05447.x
Casile, A., and Giese, M. A. (2006). Nonvisual motor training influences biological motion perception. Curr Biol. 16, 69–74. doi: 10.1016/j.cub.2005.10.071
Caspi, A., Hariri, A. R., Holmes, A., Uher, R., and Moffitt, T. E. (2010). Genetic sensitivity to the environment: the case of the serotonin transporter gene and its implications for studying complex diseases and traits. Am. J. Psychiatry 167, 509–527. doi: 10.1176/appi.ajp.2010.09101452
Caspi, A., Sugden, K., Moffitt, T. E., Taylor, A., Craig, I. W., Harrington, H., et al. (2003). Influence of life stress on depression: moderation by a polymorphism in the 5-HTT gene. Science 301, 386–389. doi: 10.1126/science.1083968
Cisek, P. (2007). Cortical mechanisms of action selection: the affordance competition hypothesis. Philos. Trans. R. Soc. B Biol. Sci. 362, 1585–1599. doi: 10.1098/rstb.2007.2054
Cisek, P., and Kalaska, J. F. (2010). Neural mechanisms for interacting with a world full of action choices. Annu. Rev. Neurosci. 33, 269–298. doi: 10.1146/annurev.neuro.051508.135409
Cisek, P., and Pastor-Bernier, A. (2014). On the challenges and mechanisms of embodied decisions. Philos. Trans. R. Soc. Lond. B Biol. Sci. 369:20130479. doi: 10.1098/rstb.2013.0479
Clark, A. (1999). An embodied cognitive science? Trends Cogn. Sci. 3, 345–351. doi: 10.1016/S1364-6613(99)01361-3
Collins, S., Ruina, A., Tedrake, R., and Wisse, M. (2005). Efficient bipedal robots based on passive dynamic walkers. Science 307, 1082–1085. doi: 10.1126/science.1107799
Cortes, L. R., Cisternas, C. D., and Forger, N. G. (2019). Does gender leave an epigenetic imprint on the brain? Front. Neurosci. 13:173. doi: 10.3389/fnins.2019.00173
Craig, A. D. B. (2004). Human feelings: why are some more aware than others? Trends Cogn. Sci. 8, 239–241. doi: 10.1016/j.tics.2004.04.004
Craig, A. D. B. (2009a). Emotional moments across time: a possible neural basis for time perception in the anterior insula. Philos. Trans. R. Soc. Lond. B Biol. Sci. 364, 1933–1942. doi: 10.1098/rstb.2009.0008
Craig, A. D. B. (2009b). How do you feel–now? The anterior insula and human awareness. Nat. Rev. Neurosci. 10, 59–70. doi: 10.1038/nrn2555
Critchley, H. D., and Garfinkel, S. N. (2018). The influence of physiological signals on cognition. Curr. Opin. Behav. Sci. 19, 13–18. doi: 10.1016/j.cobeha.2017.08.014
Critchley, H. D., Wiens, S., Rotshtein, P., Öhman, A., and Dolan, R. J. (2004). Neural systems supporting interoceptive awareness. Nat. Neurosci. 7, 189–195. doi: 10.1038/nn1176
Culverhouse, R. C., Saccone, N. L., Horton, A. C., Ma, Y., Anstey, K. J., Banaschewski, T., et al. (2018). Collaborative meta-analysis finds no evidence of a strong interaction between stress and 5-HTTLPR genotype contributing to the development of depression. Mol. Psychiatry 23, 133–142. doi: 10.1038/mp.2017.44
Damasio, A., Damasio, H., and Tranel, D. (2013). Persistence of feelings and sentience after bilateral damage of the insula. Cereb. Cortex 23, 833–846. doi: 10.1093/cercor/bhs077
Damasio, A. R. (1994). Descartes' Error: Emotion, Reason, and the Human Brain. New York, NY: Putnam.
Damasio, A. R. (1996). The somatic marker hypothesis and the possible functions of the prefrontal cortex. Philos. Trans. R. Soc. Lond. B Biol. Sci. 351, 1413–1420. doi: 10.1098/rstb.1996.0125
Damasio, A. R. (1999). The Feeling of What Happens: Body and Emotion in the Making of Consciousness. New York, NY: Houghton Mifflin Harcourt.
Danese, A., Caspi, A., Williams, B., Ambler, A., Sugden, K., Mika, J., et al. (2011). Biological embedding of stress through inflammation processes in childhood. Mol. Psychiatry 16, 244–246. doi: 10.1038/mp.2010.5
Decety, J., and Michalska, K. J. (2020). “Chapter 22—A developmental neuroscience perspective on empathy,” in Neural Circuit and Cognitive Development, 2nd Edn, eds J. Rubenstein, P. Rakic, B. Chen, and K. Y. Kwan (London: Academic Press), 485–503. doi: 10.1016/B978-0-12-814411-4.00022-6
Eilan, N. (Ed.). (2005). Joint Attention: Communication and Other Minds; Issues in Philosophy and Psychology. Consciousness and Self-Consciousness. Oxford; New York, NY: Clarendon Press.
Eppinger, B., Hämmerer, D., and Li, S. C. (2011). Neuromodulation of reward-based learning and decision making in human aging. Ann. N. Y. Acad. Sci. 1235, 1–17. doi: 10.1111/j.1749-6632.2011.06230.x
Esposito, G., Azhari, A., and Borelli, J. L. (2018). Gene × environment interaction in developmental disorders: where do we stand and what's next? Front. Psychol. 9:2036. doi: 10.3389/fpsyg.2018.02036
Fawcett, C., Arslan, M., Falck-Ytter, T., Roeyers, H., and Gredebäck, G. (2017). Human eyes with dilated pupils induce pupillary contagion in infants. Sci. Rep. 7:9601. doi: 10.1038/s41598-017-08223-3
Fawcett, C., Wesevich, V., and Gredebäck, G. (2016). Pupillary contagion in infancy: evidence for spontaneous transfer of arousal. Psychol. Sci. 27, 997–1003. doi: 10.1177/0956797616643924
Feder, A., Nestler, E. J., and Charney, D. S. (2009). Psychobiology and molecular genetics of resilience. Nat. Rev. Neurosci. 10, 446–457. doi: 10.1038/nrn2649
Filimon, F., Nelson, J. D., Hagler, D. J., and Sereno, M. I. (2007). Human cortical representations for reaching: mirror neurons for execution, observation, and imagery. Neuroimage 37, 1315–1328. doi: 10.1016/j.neuroimage.2007.06.008
Fogelman, N., and Canli, T. (2019). Early life stress, physiology, and genetics: a review. Front. Psychol. 10:1668. doi: 10.3389/fpsyg.2019.01668
Francis, D. D., Diono, J., Plotsky, P. M., and Meaney, M.J. (2002). Environmental enrichment reverses the effects of maternal separation on stress reactivity. J. Neurosci. 22, 7840–7843. doi: 10.1523/JNEUROSCI.22-18-07840.2002
Franklin, T. B., Russig, H., Weiss, I. C., Gräff, J., Linder, N., Michalon, A., et al. (2010). Epigenetic transmission of the impact of early stress across generations. Biol. Psychiatry 68, 408–415. doi: 10.1016/j.biopsych.2010.05.036
Frith, U. (2014). Autism-are we any closer to explaining the enigma? Psychologist 27, 744–745. Available online at: https://thepsychologist.bps.org.uk/volume-27/edition-10/autism-are-we-any-closer-explaining-enigma
Fuchs, T. (2008). The Brain as a Relational Organ. A Phenomenological and Ecological Concept [in German]. Stuttgart: Kohlhammer.
Fuchs, T. (2016a). “Embodied knowledge - embodied memory,” in Analytic and Continental Philosophy: Methods and Perspectives: Proceedings of the 37th International Wittgenstein Symposium, eds H. A. Wiltsche and S. Rinofner-Kreidl (Berlin: De Gruyter), 215–229. doi: 10.1515/9783110450651-015
Fuchs, T. (2016b). “The embodied development of language,” in Embodiment in Evolution and Culture, 1st Edn, eds G. Etzelmüller and C. Tewes (Tübingen: Mohr Siebeck), 107–128.
Fuchs, T. (2017). Ecology of the Brain: The Phenomenology and Biology of the Embodied Mind. Oxford: Oxford University Press. doi: 10.1093/med/9780199646883.001.0001
Gaigg, S. B., Cornell, A. S., and Bird, G. (2018). The psychophysiological mechanisms of alexithymia in autism spectrum disorder. Autism 22, 227–231. doi: 10.1177/1362361316667062
Gallagher, S. (2005). How the Body Shapes the Mind. New York, NY: Clarendon Press. doi: 10.1093/0199271941.001.0001
Gallese, V. (2007). Embodied simulation: from mirror neuron systems to interpersonal relations. Novartis Found. Symp. 278, 3–12; discussion: 12–9, 89–96, 216–21. doi: 10.1002/9780470030585.ch2
Gallese, V. (2017). Visions of the body. Embodied simulation and aesthetic experience. Aisthesis 10, 41–50. doi: 10.13128/Aisthesis-20902
Gallese, V. (2019). Embodied simulation. Its bearing on aesthetic experience and the dialogue between neuroscience and the humanities. Gestalt Theor. 41, 113–127. doi: 10.2478/gth-2019-0013
Gallese, V., and Goldman, A. (1998). Mirror neurons and the simulation theory of mind-reading. Trends Cogn. Sci. 2, 493–501. doi: 10.1016/S1364-6613(98)01262-5
Gallivan, J. P., and Chapman, C. S. (2014). Three-dimensional reach trajectories as a probe of real-time decision-making between multiple competing targets. Front. Neurosci. 8:215. doi: 10.3389/fnins.2014.00215
Gallivan, J. P., Chapman, C. S., Wolpert, D. M., and Flanagan, J. R. (2018). Decision-making in sensorimotor control. Nat. Rev. Neurosci. 19, 519–534. doi: 10.1038/s41583-018-0045-9
Garfinkel, S. N., Tiley, C., O'Keeffe, S., Harrison, N. A., Seth, A. K., and Critchley, H. D. (2016). Discrepancies between dimensions of interoception in autism: implications for emotion and anxiety. Biol. Psychol. 114, 117–126. doi: 10.1016/j.biopsycho.2015.12.003
Gibbs, R. W. (2005). Embodiment and Cognitive Science. Cambridge: Cambridge University Press. doi: 10.1017/CBO9780511805844
Gibson, J. J. (1986//2014). The Ecological Approach to Visual Perception: Classic Edition. Psychology Press and Routledge Classic Editions. Hoboken, NJ: Taylor and Francis. doi: 10.4324/9781315740218
Glenberg, A. M. (2015). Few believe the world is flat: how embodiment is changing the scientific understanding of cognition. Can. J. Exp. Psychol. 69, 165–171. doi: 10.1037/cep0000056
Glenberg, A. M., and Gallese, V. (2012). Action-based language: a theory of language acquisition, comprehension, and production. Cortex 48, 905–922. doi: 10.1016/j.cortex.2011.04.010
Gluckman, P. D., Buklijas, T., and Hanson, M. A. (2016). “Chapter 1—The developmental origins of health and disease (DOHaD) concept: past, present, and future,” in The Epigenome and Developmental Origins of Health and Disease, ed C. S. Rosenfeld (Boston, MA: Academic Press), 1–15. doi: 10.1016/B978-0-12-801383-0.00001-3
Godfrey, K. M., Lillycrop, K. A., Burdge, G. C., Gluckman, P. D., and Hanson, M. A. (2007). Epigenetic mechanisms and the mismatch concept of the developmental origins of health and disease. Pediatr. Res. 61, 5–10. doi: 10.1203/pdr.0b013e318045bedb
Goldsmith, R. E., Freyd, J. J., and DePrince, A. P. (2012). Betrayal trauma: associations with psychological and physical symptoms in young adults. J. Interpers. Violence 27, 547–567. doi: 10.1177/0886260511421672
Gottwald, J. M., Achermann, S., Marciszko, C., Lindskog, M., and Gredebäck, G. (2016). An embodied account of early executive-function development. Psychol. Sci. 27, 1600–1610. doi: 10.1177/0956797616667447
Gredebäck, G. (2018). How visual and motor experience shapes the development of infants' perception of actions performed by social partners. J. Motor Learn. Dev. 6, S89–S104. doi: 10.1123/jmld.2016-0074
Gredebäck, G., and Falck-Ytter, T. (2015). Eye movements during action observation. Perspect. Psychol. Sci. 10, 591–598. doi: 10.1177/1745691615589103
Griffiths, P. E., and Tabery, J. (2013). “Chapter 3—Developmental systems theory: what does it explain, and how does it explain it?” in Advances in Child Development and Behavior, eds R. M. Lerner and J. B. Benson (Waltham, MA: Academic Press), 65–94. doi: 10.1016/B978-0-12-397947-6.00003-9
Hagura, N., Haggard, P., and Diedrichsen, J. (2017). Perceptual decisions are biased by the cost to act. Elife 6:e18422. doi: 10.7554/eLife.18422
Hanson, M. A., and Gluckman, P. D. (2008). Developmental origins of health and disease: new insights. Basic Clin. Pharmacol. Toxicol. 102, 90–93. doi: 10.1111/j.1742-7843.2007.00186.x
Harris, M. A., Brett, C. E., Starr, J. M., Deary, I. J., and McIntosh, A. M. (2016). Early-life predictors of resilience and related outcomes up to 66 years later in the 6-day sample of the 1947 Scottish mental survey. Soc. Psychiatry Psychiatr. Epidemiol. 51, 659–668. doi: 10.1007/s00127-016-1189-4
Hartmann, J., and Schmidt, M. V. (2020). “Chapter 11—Stress resilience as a consequence of early-life adversity,” in Stress Resilience, ed A. Chen (London: Academic Press), 149–164. doi: 10.1016/B978-0-12-813983-7.00011-2
Haywood, K., and Getchell, N. (2020). Life Span Motor Development, 7th Edn. Champaign, IL: Human Kinetics.
Herbert, B. M., and Pollatos, O. (2012). The body in the mind: on the relationship between interoception and embodiment. Top. Cogn. Sci. 4, 692–704. doi: 10.1111/j.1756-8765.2012.01189.x
Hertzman, C. (2012). Putting the concept of biological embedding in historical perspective. Proc. Natl. Acad. Sci. U.S.A. 109(Suppl. 2), 17160–17167. doi: 10.1073/pnas.1202203109
Hoffman, G. (2012). Embodied cognition for autonomous interactive robots. Top. Cogn. Sci. 4, 759–772. doi: 10.1111/j.1756-8765.2012.01218.x
Hoffmann, M., and Pfeifer, R. (2018). “Robots as powerful allies for the study of embodied cognition from the bottom up,” in The Oxford Handbook 4e Cognition, eds A. Newen, L. de Bruin, and S. Gallagher (Oxford: Oxford University Press), 841–862. doi: 10.1093/oxfordhb/9780198735410.013.45
Hornecker, E., Marshall, P., and Hurtienne, J. (2017). Locating Theories of Embodiment Along Three Axes: 1st-3rd Person, Body-Context, Practice-Cognition. Retrieved from: https://www.semanticscholar.org/paper/1-Locating-Theories-of-Embodiment-Along-Three-Axes-Hornecker/e4a11294dbdb71317d62a4f1e9a5ac8961d839c1 (accessed July 07, 2021).
Horsthemke, B. (2018). A critical view on transgenerational epigenetic inheritance in humans. Nat. Commun. 9:2973. doi: 10.1038/s41467-018-05445-5
Hunter, D. J. (2005). Gene–environment interactions in human diseases. Nat. Rev. Genet. 6, 287–298. doi: 10.1038/nrg1578
Huys, Q. J., Maia, T. V., and Frank, M. J. (2016). Computational psychiatry as a bridge from neuroscience to clinical applications. Nat. Neurosci. 19:404. doi: 10.1038/nn.4238
IJzerman, H., and Semin, G. R. (2010). Temperature perceptions as a ground for social proximity. J. Exp. Soc. Psychol. 46, 867–873. doi: 10.1016/j.jesp.2010.07.015
Inhelder, B., and Piaget, J. (1958). The Growth of Logical Thinking From Childhood to Adolescence: An essay on the Construction of Formal Operational Strucutres. London; New York, NY: Routledge Taylor and Francis Group. doi: 10.1037/10034-000
Inkster, M., Wellsby, M., Lloyd, E., and Pexman, P. M. (2016). Development of embodied word meanings: sensorimotor effects in children's lexical processing. Front. Psychol. 7:317. doi: 10.3389/fpsyg.2016.00317
James, W. (1994). The physical basis of emotion. Psychol. Rev. 101, 205–210. doi: 10.1037/0033-295X.101.2.205
Kamm, K., Thelen, E., and Jensen, J. L. (1990). A dynamical systems approach to motor development. Phys. Ther. 70, 763-775. doi: 10.1093/ptj/70.12.763
Kang, Y., Williams, L. E., Clark, M. S., Gray, J. R., and Bargh, J. A. (2011). Physical temperature effects on trust behavior: the role of insula. Soc. Cogn. Affect. Neurosci. 6, 507–515. doi: 10.1093/scan/nsq077
Khalsa, S. S., Adolphs, R., Cameron, O. G., Critchley, H. D., Davenport, P. W., Feinstein, J. S., et al. (2018). Interoception and mental health: a roadmap. Biol. Psychiatry Cogn. Neurosci. Neuroimaging 3, 501–513. doi: 10.1016/j.bpsc.2018.04.007
Kilner, J. M., Friston, K. J., and Frith, C. D. (2007). Predictive coding: an account of the mirror neuron system. Cogn. Process. 8, 159–166. doi: 10.1007/s10339-007-0170-2
Kirmayer, L. J., and Gómez-Carrillo, A. (2019). Agency, embodiment and enactment in psychosomatic theory and practice. Med. Humanit. 45:169. doi: 10.1136/medhum-2018-011618
Kirsch, L. P., Urgesi, C., and Cross, E. S. (2016). Shaping and reshaping the aesthetic brain: Emerging perspectives on the neurobiology of embodied aesthetics. Neurosci. Biobehav. Rev. 62, 56–68. doi: 10.1016/j.neubiorev.2015.12.005
Kiverstein, J. (2012). The meaning of embodiment. Top. Cogn. Sci. 4, 740–758. doi: 10.1111/j.1756-8765.2012.01219.x
Koch, S., Holland, R. W., and van Knippenberg, A. (2008). Regulating cognitive control through approach-avoidance motor actions. Cognition 109, 133–142. doi: 10.1016/j.cognition.2008.07.014
Körner, A., Topolinski, S., and Strack, F. (2015). Routes to embodiment. Front. Psychol. 6:940. doi: 10.3389/fpsyg.2015.00940
Krieger, N. (2005). Embodiment: a conceptual glossary for epidemiology. J. Epidemiol. Commun. Health 59, 350–355. doi: 10.1136/jech.2004.024562
Krüger, M., Eggert, T., and Straube, A. (2013). Age-related differences in the stabilization of important task variables in reaching movements. Motor Control 17, 313–319. doi: 10.1123/mcj.17.3.313
Krüger, M., and Hermsdörfer, J. (2019). Target uncertainty during motor decision-making: the time course of movement variability reveals the effect of different sources of uncertainty on the control of reaching movements. Front. Psychol. 10:41. doi: 10.3389/fpsyg.2019.00913
Kuan, P.-F., Waszczuk, M. A., Kotov, R., Marsit, C. J., Guffanti, G., Gonzalez, A., et al. (2017). An epigenome-wide DNA methylation study of PTSD and depression in World Trade Center responders. Transl. Psychiatry 7, e1158–e1158. doi: 10.1038/s41398-017-0050-1
Kuehn, E., Perez-Lopez, M. B., Diersch, N., Döhler, J., Wolbers, T., and Riemer, M. (2018). Embodiment in the aging mind. Neurosci. Biobehav. Rev. 86, 207–225. doi: 10.1016/j.neubiorev.2017.11.016
Kurnianingsih, Y. A., Sim, S. K., Chee, M. W., and Mullette-Gillman, O. (2015). Aging and loss decision making: increased risk aversion and decreased use of maximizing information, with correlated rationality and value maximization. Front. Hum. Neurosci. 9:280. doi: 10.3389/fnhum.2015.00280
Lacal, I., and Ventura, R. (2018). Epigenetic inheritance: concepts, mechanisms and perspectives. Front. Mol. Neurosci. 11:292. doi: 10.3389/fnmol.2018.00292
Lea, A. J., Vockley, C. M., Johnston, R. A., Del Carpio, C. A., Barreiro, L. B., Reddy, T. E., et al. (2018). Genome-wide quantification of the effects of DNA methylation on human gene regulation. Elife 7:e37513. doi: 10.7554/eLife.37513.046
Leitan, N. D., and Chaffey, L. (2014). Embodied cognition and its applications: a brief review. Sensoria J. Mind Brain Cult. 10, 3–10. doi: 10.7790/sa.v10i1.384
Li, S. (2006). “Biocultural co-construction of lifespan development,” in Lifespan Development and the Brain: The Perspective of Biocultural Co-Constructivism, eds F. Rösler, P. B. Baltes, and P. A. Reuter-Lorenz (Cambridge: Cambridge University Press), 40–57.
Lickliter, R., and Honeycutt, H. (2003). Developmental dynamics: toward a biologically plausible evolutionary psychology. Psychol. Bull. 129, 819–835. doi: 10.1037/0033-2909.129.6.819
Lotze, M., and Halsband, U. (2006). Motor imagery. J. Physiol. 99, 386–395. doi: 10.1016/j.jphysparis.2006.03.012
Loucks, J., and Sommerville, J. (2018). Developmental change in action perception: is motor experience the cause? Infancy 23, 519–537. doi: 10.1111/infa.12231
Lund, T. C., Sidhu, D. M., and Pexman, P. M. (2019). Sensitivity to emotion information in children's lexical processing. Cognition 190, 61–71. doi: 10.1016/j.cognition.2019.04.017
Lupien, S. J., McEwen, B. S., Gunnar, M. R., and Heim, C. (2009). Effects of stress throughout the lifespan on the brain, behaviour and cognition. Nat. Rev. Neurosci. 10, 434–445. doi: 10.1038/nrn2639
Lux, V. (2018). Epigenetic programming effects of early life stress: a dual-activation hypothesis. Curr. Genomics 19, 638–652. doi: 10.2174/1389202919666180307151358
MacLachlan, M. (2004). Embodiment: Clinical, Critical and Cultural Perspectives on Health and Illness (Health Psychology). Berkshire: Open University Press.
Manzoni, C., Kia, D. A., Vandrovcova, J., Hardy, J., Wood, N. W., Lewis, P. A., et al. (2016). Genome, transcriptome and proteome: the rise of omics data and their integration in biomedical sciences. Brief. Bioinformatics 19, 286–302. doi: 10.1093/bib/bbw114
Marasco, P. D., Kim, K., Colgate, J. E., Peshkin, M. A., and Kuiken, T. A. (2011). Robotic touch shifts perception of embodiment to a prosthesis in targeted reinnervation amputees. Brain 134, 747–758. doi: 10.1093/brain/awq361
Masten, A. S., and Cicchetti, D. (2010). Developmental cascades. Dev. Psychopathol. 22, 491–495. doi: 10.1017/S0954579410000222
Mata, R., Schooler, L. J., and Rieskamp, J. (2007). The aging decision maker: cognitive aging and the adaptive selection of decision strategies. Psychol. Aging 22:796. doi: 10.1037/0882-7974.22.4.796
McDade, T. W., Ryan, C. P., Jones, M. J., Hoke, M. K., Borja, J., Miller, G. E., et al. (2019). Genome-wide analysis of DNA methylation in relation to socioeconomic status during development and early adulthood. Am. J. Phys. Anthropol. 169, 3–11. doi: 10.1002/ajpa.23800
McGeer, T. (1990). Passive dynamic walking. Int. J. Rob. Res. 9, 62–82. doi: 10.1177/027836499000900206
Meier, B. P., Schnall, S., Schwarz, N., and Bargh, J. A. (2012). Embodiment in social psychology. Top. Cogn. Sci. 4, 705–716. doi: 10.1111/j.1756-8765.2012.01212.x
Meteyard, L., Rodriguez Cuadrado, S., Bahrami, B., and Vigliocco, G. (2012). Coming of age: a review of embodiment and the neuroscience of semantics. Cortex 48, 788–804. doi: 10.1016/j.cortex.2010.11.002
Meugnot, A., Almecija, Y., and Toussaint, L. (2014). The embodied nature of motor imagery processes highlighted by short-term limb immobilization. Exp. Psychol. 61, 180–186. doi: 10.1027/1618-3169/a000237
Moore, C., Dunham, P. J., and Dunham, P. (Eds.). (1995). Joint Attention: Its Origins and Role in Development. Hoboken, NJ: Taylor and Francis.
Mulder, R. H., Walton, E., Neumann, A., Houtepen, L. C., Felix, J. F., Bakermans-Kranenburg, M. J., et al. (2020). Epigenomics of being bullied: changes in DNA methylation following bullying exposure. Epigenetics 15, 750–764. doi: 10.1080/15592294.2020.1719303
Munzert, J., Lorey, B., and Zentgraf, K. (2009). Cognitive motor processes: the role of motor imagery in the study of motor representations. Brain Res. Rev. 60, 306–326. doi: 10.1016/j.brainresrev.2008.12.024
Murgatroyd, C., Patchev, A. V., Wu, Y., Micale, V., Bockmühl, Y., Fischer, D., et al. (2009). Dynamic DNA methylation programs persistent adverse effects of early-life stress. Nat. Neurosci. 12, 1559–1566. doi: 10.1038/nn.2436
Murphy, J., Brewer, R., Catmur, C., and Bird, G. (2017). Interoception and psychopathology: a developmental neuroscience perspective. Dev. Cogn. Neurosci. 23, 45–56. doi: 10.1016/j.dcn.2016.12.006
Needham, A., and Libertus, K. (2011). Embodiment in early development. Wiley Interdiscip. Rev. Cogn. Sci. 2, 117–123. doi: 10.1002/wcs.109
Needham, B. L., Smith, J. A., Zhao, W., Wang, X., Mukherjee, B., Kardia, S. L. R., et al. (2015). Life course socioeconomic status and DNA methylation in genes related to stress reactivity and inflammation: the multi-ethnic study of atherosclerosis. Epigenetics 10, 958–969. doi: 10.1080/15592294.2015.1085139
Nelson, C. A. (2017). Hazards to early development: the biological embedding of early life adversity. Neuron 96, 262–266. doi: 10.1016/j.neuron.2017.09.027
Newell, K. M. (1986). “Constraints on the development of coordination,” in eds NATO ASI series D, Behavioural and Social Sciences: Vol. 34. Motor Development in Children: Aspects of Coordination and Control; [Proceedings of the NATO Advanced Study Institute on Motor Skill Acquisition in Children, H. Whiting and M. G. Wade (Maastrich; Dordrecht: Nijhoff), 341–360.
Nicholson, T., Williams, D., Carpenter, K., and Kallitsounaki, A. (2019). Interoception is impaired in children, but not adults, with autism spectrum disorder. J. Autism Dev. Disord. 49, 3625-3637. doi: 10.1007/s10803-019-04079-w
Noel, J. P., Lytle, M., Cascio, C., and Wallace, M. T. (2018). Disrupted integration of exteroceptive and interoceptive signaling in autism spectrum disorder. Autism Res. 11, 194-205. doi: 10.1002/aur.1880
Non, A. L. (2021). Social epigenomics: are we at an impasse?. Epigenomics. doi: 10.2217/epi-2020-0136
Non, A. L., Hollister, B. M., Humphreys, K. L., Childebayeva, A., Esteves, K., Zeanah, C. H., et al. (2016). DNA methylation at stress-related genes is associated with exposure to early life institutionalization. Am. J. Phys. Anthropol. 161, 84–93. doi: 10.1002/ajpa.23010
Overton, W. F. (1991). “Competence, procedures, and hardware: conceptual and empirical considerations,” in Criteria for Competence: Controversies in the Conceptualization and Assessment of Children's Abilities, eds M. Chandler and M. Chapman (Hillsdale, NJ: Lawrence Erlbaum Associates, Inc.), 19–42.
Overton, W. F. (2008). “Embodiment from a relational perspective,” in The Jean Piaget Symposium Series. Developmental Perspectives on Embodiment and Consciousness, eds W. F. Overton, U. Mueller, and J. L. Newman (Hove: Psychology), 1–18. doi: 10.4324/9780203809778
Overton, W. F., and Lerner, R. M. (2012). Relational developmental systems: a paradigm for developmental science in the postgenomic era. Behav. Brain Sci. 35, 375–376. doi: 10.1017/S0140525X12001082
Overton, W. F., Müller, U., and Newman, J. L. (2008). Developmental Perspectives on Embodiment and Consciousness. The Jean Piaget Symposium Series. Hove: Psychology Press.
Oyama, S. (2000). Evolution's Eye: A Systems View of the Biology-Culture Divide. Science and Cultural Theory. Durham, NC: Duke University Press. doi: 10.1215/9780822380658
Palser, E. R., Fotopoulou, A., Pellicano, E., and Kilner, J. M. (2018). The link between interoceptive processing and anxiety in children diagnosed with autism spectrum disorder: extending adult findings into a developmental sample. Biol. Psychol. 136, 13–21. doi: 10.1016/j.biopsycho.2018.05.003
Paulus, M. P., and Stein, M. B. (2010). Interoception in anxiety and depression. Brain Struct. Funct. 214, 451–463. doi: 10.1007/s00429-010-0258-9
Perez, M. F., and Lehner, B. (2019). Intergenerational and transgenerational epigenetic inheritance in animals. Nat. Cell Biol. 21, 143–151. doi: 10.1038/s41556-018-0242-9
Petzschner, F. H., Garfinkel, S. N., Paulus, M. P., Koch, C., and Khalsa, S. S. (2021). Computational models of interoception and body regulation. Trends Neurosci. 44, 63–76. doi: 10.1016/j.tins.2020.09.012
Petzschner, F. H., Weber, L. A., Gard, T., and Stephan, K. E. (2017). Computational psychosomatics and computational psychiatry: toward a joint framework for differential diagnosis. Biol. Psychiatry 82, 421–430. doi: 10.1016/j.biopsych.2017.05.012
Pexman, P. M. (2019). The role of embodiment in conceptual development. Lang. Cogn. Neurosci. 34, 1274–1283. doi: 10.1080/23273798.2017.1303522
Pfeifer, R., and Bongard, J. (2006). How the Body Shapes the Way We Think: A New View of Intelligence. Cambridge, MA: MIT Press. doi: 10.7551/mitpress/3585.001.0001
Piaget, J. (1977). “The role of action in the development of thinking,” in Knowledge and Development, Volume 1 Advances in Research and Theory, eds W. F. Overton, and J. M. Gallagher (Boston, MA: Springer US), 17–42.
Pluess, M. (2015). Individual differences in environmental sensitivity. Child Dev. Perspect. 9, 138–143. doi: 10.1111/cdep.12120
Pluess, M., and Belsky, J. (2010). Children's differential susceptibility to effects of parenting. Fam. Sci. 1, 14–25. doi: 10.1080/19424620903388554
Ponari, M., Norbury, C. F., and Vigliocco, G. (2017). Acquisition of abstract concepts is influenced by emotional valence. Dev. Sci. 21:e12549. doi: 10.1111/desc.12549
Ponari, M., Norbury, C. F., and Vigliocco, G. (2020). The role of emotional valence in learning novel abstract concepts. Dev. Psychol. 56, 1855–1865. doi: 10.1037/dev0001091
Prinz, W. (1997). Perception and action planning. Eur. J. Cogn. Psychol. 9, 129–154. doi: 10.1080/713752551
Provençal, N., Arloth, J., Cattaneo, A., Anacker, C., Catane, N., Wiechmann, T., et al. (2019). Glucocorticoid exposure during hippocampal neurogenesis primes future stress response by inducing changes in DNA methylation. Proc. Natl. Acad. Sci. U.S.A. 117, 23280–23285. doi: 10.1073/pnas.1820842116
Provençal, N., and Binder, E. (2014). The effects of early life stress on the epigenome: from the womb to adulthood and even before. Exp. Neurol. 268, 10–20. doi: 10.1016/j.expneurol.2014.09.001
Ptak, R., Schnider, A., and Fellrath, J. (2017). The dorsal frontoparietal network: a core system for emulated action. Trends Cogn. Sci. 21, 589–599. doi: 10.1016/j.tics.2017.05.002
Pylyshyn, Z. W. (1985). Computation and Cognition: Toward a Foundation for Cognitive Science, 2nd Edn. London: MIT Press. doi: 10.7551/mitpress/2004.001.0001
Quattrocki, E., and Friston, K. (2014). Autism, oxytocin and interoception. Neurosci. Biobehav. Rev. 47, 410-430. doi: 10.1016/j.neubiorev.2014.09.012
Quillian, M.R. (1969). The teachable language comprehender: a simulation program and theory of language. Commun. ACM 12, 459-476. doi: 10.1145/363196.363214
Ramo-Fernández, L., Schneider, A., Wilker, S., and Kolassa, I.-T. (2015). Epigenetic alterations associated with war trauma and childhood maltreatment. Behav. Sci. Law 33, 701–721. doi: 10.1002/bsl.2200
Redish, A. D., and Gordon, J. A. (Eds.). (2016). Computational Psychiatry: New Perspectives on Mental Illness, Vol. 20. Cambridge, MA: MIT Press. doi: 10.7551/mitpress/9780262035422.001.0001
Risch, N., Herrell, R., Lehner, T., Liang, K.-Y., Eaves, L., Hoh, J., et al. (2009). Interaction between the serotonin transporter gene (5-HTTLPR), stressful life events, and risk of depression: a meta-analysis. JAMA 301, 2462–2471. doi: 10.1001/jama.2009.878
Rizzolatti, G., and Arbib, M. A. (1998). Language within our grasp. Trends Neurosci. 21, 188–194. doi: 10.1016/S0166-2236(98)01260-0
Rizzolatti, G., and Craighero, L. (2004). The mirror-neuron system. Annu. Rev. Neurosci. 27, 169–192. doi: 10.1146/annurev.neuro.27.070203.144230
Rizzolatti, G., Fadiga, L., Gallese, V., and Fogassi, L. (1996). Premotor cortex and the recognition of motor actions. Brain Res. Cogn. Brain Res. 3, 131–141. doi: 10.1016/0926-6410(95)00038-0
Roh, J. W., Park, H. J., and Kang, U. G. (2007). Hormones and psychiatric disorders. Clin. Psychopharmacol. Neurosci. 5, 3–13. Available online at: https://www.cpn.or.kr/journal/view.html?uid=42&vmd=Full
Rutter, M. (2012). Achievements and challenges in the biology of environmental effects. Proc. Natl. Acad. Sci. U.S.A. 109(Suppl. 2):17149. doi: 10.1073/pnas.1121258109
Rutter, M. (2016). Why is the topic of the biological embedding of experiences important for translation? Dev. Psychopathol. 28(4 Pt 2), 1245–1258. doi: 10.1017/S0954579416000821
Salzer, Y., and Friedman, J. (2019). Reaching trajectories unravel modality-dependent temporal dynamics of the automatic process in the Simon task: a model-based approach. Psychol. Res. 82, 734–743. doi: 10.1007/s00426-019-01177-3
Schmuckler, M. A. (1993). “Perception-action coupling in infancy,” in The Development of Coordination in Infancy. Advances in Psychology, Vol. 97, ed. G. J. P. Savelsbergh (Amsterdam: North-Holland), 137–173. doi: 10.1016/S0166-4115(08)60952-8
Schnurr, P. P., Spiro, A. III, Aldwin, C. M., and Stuekl, T. A. (1998). Physical symptom trajectories following trauma exposure: longitudinal findings from the normative aging study. J Nerv Ment Dis. 186, 522–528. doi: 10.1097/00005053-199809000-00002
Schöner, G., Tekülve, J., and Zibner, S. (2018). “Reaching for objects: a neural process account in a developmental perspective,” in Reach-to-Grasp Behavior: Brain, Behavior and Modelling Across the Life Span, eds D. Corbetta and M. Santello (New York, NY: Routledge), 281–318. doi: 10.4324/9780429467875-12
Sebanz, N., Bekkering, H., and Knoblich, G. (2006). Joint action: bodies and minds moving together. Trends Cogn. Sci. 10, 70–76. doi: 10.1016/j.tics.2005.12.009
Seligman, R., Choudhury, S., and Kirmayer, L. J. (2015). “Locating culture in the brain and in the world: from social categories to an ecology of mind,” in Oxford Library of Psychology. The Oxford Handbook of Cultural Neuroscience, eds J. Y. Chiao, S.-C. Li, R. Seligman, and R. Turner (New York, NY; Oxford; Auckland: Oxford University Press), 3–20. doi: 10.1093/oxfordhb/9780199357376.013.3
Serpeloni, F., Radtke, K. M., Hecker, T., Sill, J., Vukojevic, V., de Assis, S. G., et al. (2019). Does prenatal stress shape postnatal resilience? An epigenome-wide study on violence and mental health in humans. Front. Genet. 10:269. doi: 10.3389/fgene.2019.00269
Seth, A. K. (2013). Interoceptive inference, emotion, and the embodied self. Trends Cogn. Sci. 17, 565–573. doi: 10.1016/j.tics.2013.09.007
Seth, A. K., and Friston, K. J. (2016). Active interoceptive inference and the emotional brain. Philos. Trans. R. Soc. B Biol. Sci. 371:20160007. doi: 10.1098/rstb.2016.0007
Shah, P., Hall, R., Catmur, C., and Bird, G. (2016). Alexithymia, not autism, is associated with impaired interoception. Cortex 81, 215–220. doi: 10.1016/j.cortex.2016.03.021
Shapiro, L. (2014). The Routledge Handbook of Embodied Cognition. Routledge Handbooks in Philosophy. New York, NY: Routledge/Taylor and Francis Group. doi: 10.4324/9781315775845
Sidhu, D. M., and Pexman, P. M. (2016). Is moving more memorable than proving? Effects of embodiment and imagined enactment on verb memory. Front. Psychol. 7:1010. doi: 10.3389/fpsyg.2016.01010
Sloman, A. (2009). “Some requirements for human-like robots: why the recent over-emphasis on embodiment has held up progress,” in Creating Brain-Like Intelligence. Lecture Notes in Computer Science, Vol. 5436, eds B. Sendhoff, E. Körner, O. Sporns, H. Ritter and K. Doya (Berlin; Heidelberg: Springer), 248–277. doi: 10.1007/978-3-642-00616-6_12
Soares, J. M., Magalhães, R., Moreira, P. S., Sousa, A., Ganz, E., Sampaio, A., et al. (2016). A Hitchhiker's guide to functional magnetic resonance imaging. Front. Neurosci. 10:515. doi: 10.3389/fnins.2016.00515
Staudinger, U. M., and Baltes, P. B. (2001). “Lifespan developmental psychology,” in Contemporary Psychiatry, eds F. Henn, N. Sartorius, H. Helmchen, and H. Lauter (Berlin; Heidelberg: Springer; Imprint), 751–759. doi: 10.1007/978-3-642-59519-6_43
Stephan, K. E., Manjaly, Z. M., Mathys, C. D., Weber, L. A., Paliwal, S., Gard, T., et al. (2016). Allostatic self-efficacy: a metacognitive theory of dyshomeostasis-induced fatigue and depression. Front. Hum. Neurosci. 10:550. doi: 10.3389/fnhum.2016.00550
Tekülve, J., Fois, A., Sandamirskaya, Y., and Schöner, G. (2019). Autonomous sequence generation for a neural dynamic robot: scene perception, serial order, and object-oriented movement. Front. Neurorobot. 13:95. doi: 10.3389/fnbot.2019.00095
Thelen, E. (2000). Grounded in the world: developmental origins of the embodied mind. Infancy 1, 3–28. doi: 10.1207/S15327078IN0101_02
Thelen, E., Kelso, J. S., and Fogel, A. (1987). Self-organizing systems and infant motor development. Dev. Rev. 7, 39–65. doi: 10.1016/0273-2297(87)90004-9
Thompson, E. (2007). Mind in Life: Biology, Phenomenology, and the Sciences of Mind. Cambridge: Harvard University Press.
Toyama, M., and Fuller, H. R. (2020). Longitudinal stress-buffering effects of social integration for late-life functional health. Int. J. Aging Hum. Dev. 91, 501–519. doi: 10.1177/0091415019871196
Tyers, M., and Mann, M. (2003). From genomics to proteomics. Nature 422, 193–197. doi: 10.1038/nature01510
Vaiserman, A. M. (2015). Epigenetic programming by early-life stress: evidence from human populations. Dev. Dyn. 244, 254–265. doi: 10.1002/dvdy.24211
Varela, F. J., Thompson, E., and Rosch, E. (1991). The Embodied Mind: Cognitive Science and Human Experience. Cambridge, MA: MIT Press. doi: 10.7551/mitpress/6730.001.0001
Vermeulen, N., and Mermillod, M. (2010). Fast emotional embodiment can modulate sensory exposure in perceivers. Commun. Integr. Biol. 3, 184–187. doi: 10.4161/cib.3.2.10922
Verrel, J., Lövdén, M., and Lindenberger, U. (2012). Normal aging reduces motor synergies in manual pointing. Neurobiol. Aging 33, 200.e1–200.e10. doi: 10.1016/j.neurobiolaging.2010.07.006
Vesper, C., Butterfill, S., Knoblich, G., and Sebanz, N. (2010). A minimal architecture for joint action. Neural Netw. 23, 998–1003. doi: 10.1016/j.neunet.2010.06.002
Vitrinel, B., Koh, H. W. L., Mujgan Kar, F., Maity, S., Rendleman, J., Choi, H., et al. (2019). Exploiting interdata relationships in next-generation proteomics analysis. Mol. Cell. Proteomics 18(8 Suppl. 1), S5–S14. doi: 10.1074/mcp.MR118.001246
von Hofsten, C. (2004). An action perspective on motor development. Trends Cogn. Sci. 8, 266–272. doi: 10.1016/j.tics.2004.04.002
Wadhwa, P. D., Buss, C., Entringer, S., and Swanson, J. M. (2009). Developmental origins of health and disease: brief history of the approach and current focus on epigenetic mechanisms. Semin. Reprod. Med. 27, 358–368. doi: 10.1055/s-0029-1237424
Weaver, I. C. G., Cervoni, N., Champagne, F. A., D'Alessio, A. C., Sharma, S., Seckl, J. R., et al. (2004). Epigenetic programming by maternal behavior. Nat. Neurosci. 7, 847–854. doi: 10.1038/nn1276
Wellsby, M., and Pexman, P. M. (2014). Developing embodied cognition: Insights from children's concepts and language processing. Front. Psychol. 5:506. doi: 10.3389/fpsyg.2014.00506
Wenger, E., Brozzoli, C., Lindenberger, U., and Lövdén, M. (2017). Expansion and renormalization of human brain structure during skill acquisition. Trends Cogn. Sci. 21, 930–939. doi: 10.1016/j.tics.2017.09.008
Wilson, M. (2002). Six views of embodied cognition. Psych. Bull. Rev. 9, 625–636. doi: 10.3758/BF03196322
Yuan, N., Chen, Y., Xia, Y., Dai, J., and Liu, C. (2019). Inflammation-related biomarkers in major psychiatric disorders: a cross-disorder assessment of reproducibility and specificity in 43 meta-analyses. Transl. Psychiatry 9:233. doi: 10.1038/s41398-019-0570-y
Zannas, A. S., Arloth, J., Carrillo-Roa, T., Iurato, S., Röh, S., Ressler, K. J., et al. (2015). Lifetime stress accelerates epigenetic aging in an urban, African American cohort: relevance of glucocorticoid signaling. Genome Biol. 16:266. doi: 10.1186/s13059-015-0828-5
Zdrazilova, L., Sidhu, D. M., and Pexman, P. M. (2018). Communicating abstract meaning: Concepts revealed in words and gestures. Philos. Trans. R. Soc. Lond. B Biol. Sci. 373:20170138. doi: 10.1098/rstb.2017.0138
Zhang, T.-Y., Keown, C. L., Wen, X., Li, J., Vousden, D. A., Anacker, C., et al. (2018). Environmental enrichment increases transcriptional and epigenetic differentiation between mouse dorsal and ventral dentate gyrus. Nat. Commun. 9:298. doi: 10.1038/s41467-017-02748-x
Zhang, T.-Y., and Meaney, M. J. (2009). Epigenetics and the environmental regulation of the genome and its function. Annu. Rev. Psychol. 61, 439–466. doi: 10.1146/annurev.psych.60.110707.163625
Keywords: embodied experiences, agency approach, environmental approach, developmental systems theory, language acquisition, cognition, perception, interoception
Citation: Lux V, Non AL, Pexman PM, Stadler W, Weber LAE and Krüger M (2021) A Developmental Framework for Embodiment Research: The Next Step Toward Integrating Concepts and Methods. Front. Syst. Neurosci. 15:672740. doi: 10.3389/fnsys.2021.672740
Received: 26 February 2021; Accepted: 28 June 2021;
Published: 30 July 2021.
Edited by:
Dietmar Plenz, National Institute of Mental Health, National Institutes of Health (NIH), United StatesReviewed by:
Bigna Lenggenhager, University of Zurich, SwitzerlandClaudio Castellini, Helmholtz Association of German Research Centers (HZ), Germany
Copyright © 2021 Lux, Non, Pexman, Stadler, Weber and Krüger. This is an open-access article distributed under the terms of the Creative Commons Attribution License (CC BY). The use, distribution or reproduction in other forums is permitted, provided the original author(s) and the copyright owner(s) are credited and that the original publication in this journal is cited, in accordance with accepted academic practice. No use, distribution or reproduction is permitted which does not comply with these terms.
*Correspondence: Vanessa Lux, vanessa.lux@rub.de